Part of a series of articles titled Alaska Park Science - Volume 17, Issue 1. Migration: On the Move in Alaska.
Article
Migration's Foundation: Ecological Intactness of Alaska's Ecosystems
E. Jamie Trammell, Southern Oregon University
Jason J. Taylor, National Park Service

Actors upon a stage, aptly describes the relation-ship between migratory species and the landscapes through which migration occurs.
Migrating species biannually travel from breeding grounds to wintering grounds, sometimes across great distances. Many factors influence the success of a migration—habitat quality on breeding and wintering grounds, ample time to accumulate energy reserves needed to make the trip, and a suitable landscape across which migration can occur with minimal hindrances to movement (we use landscape herein because we will focus on terrestrial systems and non-avian migrations, but the general concepts equally apply to seascapes, airscapes, and aquatic systems). The more difficult it is to move through landscapes, the more energy reserves are expended and the less likely migration will be successful. This makes understanding landscape permeability very important. Landscape permeability, usually defined as “the quality of a … landscape to provide passage of animals,” provides “…a broad measure of resistance to animal movement…” (Singleton 2002:2). Permeability differs by species and their sensitivity to specific hindrances (human activity, for example), by locomotion strategy (e.g., wind dispersal versus walking, flying, or swimming), and other factors. While these factors are important for species-specific management, our interest here is not to describe the permeability of landscapes at the species level, but to provide an overall assessment of landscape permeability on a sub-continental scale—the entire state of Alaska.
In more developed regions, including across much of the lower-48 United States, landscapes are highly fragmented in comparison to the sparsely populated northern latitudes. Fragmentation, due to urbanization (e.g., building highways, industrial roads, and shopping malls) or land-use conversion (e.g., forests and prairies becoming cornfields or golf courses), increases resistance to movement and decreases permeability for many species. A recent review of movement data for 57 mammal species found that in areas with a relatively high human footprint (more fragmented) a species’ extent of movement was reduced by one half to one third on average compared to areas with very low human footprint (more intact). This was due, in part, to the loss of species with long-range movements no longer found in areas with higher human impact (Tucker et al. 2018). Such changes in movement patterns and species composition impact species themselves, the region’s ecology, as well as human users who may no longer be able to access a species (e.g., decreased hunting opportunity).
In fragmented systems, conservation biologists and planners typically use the patch-corridor-matrix model of landscape ecology (Forman 1995) for assessing and restoring habitat connectivity. In this conservation model, patches are isolated areas of habitat, corridors are landscape connectivity features that support movement of species between patches, and the matrix is a heterogeneous background with various levels of resistance to movement, often relatively inhospitable to focal species. Attempts to ensure migration opportunity typically focus on connectivity between patches of similar habitats on a local scale, or between conservation areas regionally. For decades, most landscape conservation attention has been devoted to enhancing migratory capacity in the continent’s mid-latitudes by re-connecting fragmented landscapes (e.g., Belote et al. 2017). Without the appropriate connectivity, a landscape becomes impermeable (where “appropriate” depends on the targeted species).
In large, relatively unfragmented landscapes, as commonly found in northern latitudes around the globe, assessments of connectivity are less inform-ative than assessments of landscape intactness. The very limited human-development footprint is isolated to relatively small patches in a matrix of heterogeneous, contiguous habitat across large geographies (on the order of many hundreds of miles in all directions). At these broad scale, metrics of landscape intactness—“quantifiable estimate[s] of naturalness measured on a gradient of anthro-pogenic influence across broad landscapes or ecoregions” (Carter et al. 2017:55) are thus also informative about landscape permeability.
Intactness is an indicator of “the absence of human modification of the habitat” (Theobald 2013: 1859). Landscapes with high levels of intactness are considered to have higher retention of (historical) ecological structure, composition, and function (Hak and Comer 2017). In other words, highly intact regions are also commonly assumed to have high ecological integrity (Theobald 2013).
Here we summarize recent work providing the first quantitative assessment of intactness for Alaska as a whole and for each of its 32 ecoregions (Figure 1) as defined by Nowacki and colleagues (2001). This assessment provides a quantitative foundation for discussions of landscape-scale planning and management in Alaska, as well as highlights the potential for a more detailed assessment of climate connectivity (McGuire et al. 2016). We also discuss the conceptual limitations of intactness metrics in their failure to capture major climate-driven changes in landform and ecological processes in northern systems.
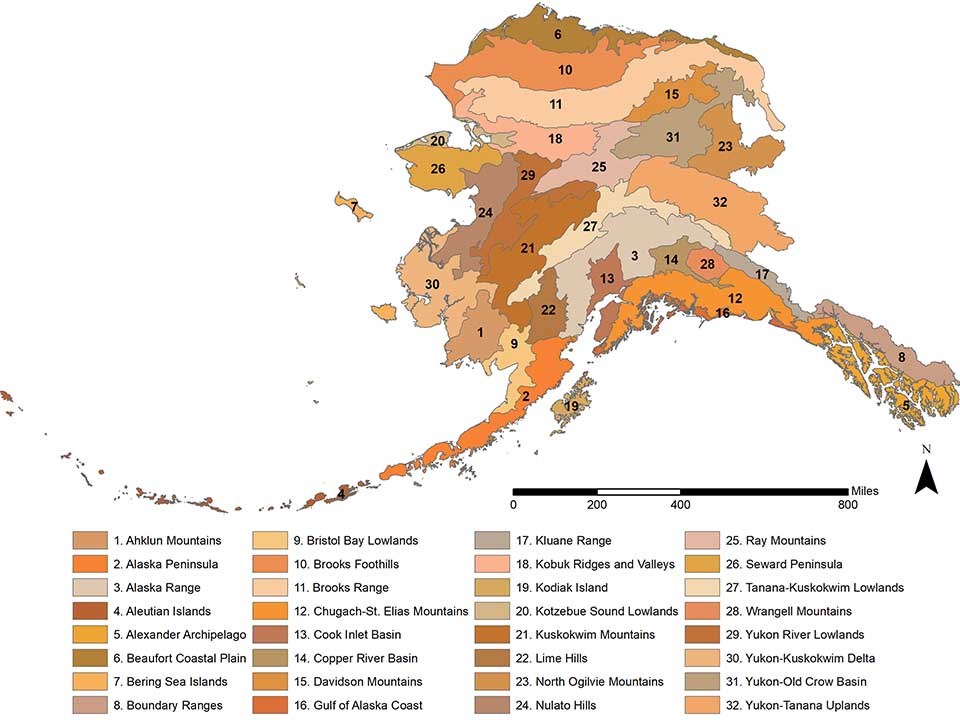
The Intactness Metric
Landscape intactness was assessed using the Landscape Condition Model (LCM) methodology (Hak and Comer 2017) modified to better represent the effect of human development on systems in Alaska (Trammell and Aisu 2015). The LCM measures intactness by first quantifying the direct impact (called the impact score) and the indirect impact (called the decay distance) of each of a variety of types of human modifications on the landscape then combining the resulting scores into a single measure of intactness at each pixel or grid cell.
Each type of human footprint is assigned a site impact score that varies from 0.05 for major highways and high-density urban development to 0.9 for areas recently logged but returning to natural forest. These impact scores are a relative measure; they are not focused on any particular ecological resource (species or habitat), but rather represent a scaled measure of the overall impact of a human activity on the landscape. Values range from 0 (lowest condition landscapes, limited function) to 1 (highest condition landscapes, fully functioning).
Using a geographic information system (GIS), these direct and indirect impacts are combined to calculate an intactness score to each 0.3 mile x 0.3 mile cell (500 meter on a side; one-quarter km2) of a statewide grid, creating a continuous map of landscape condition (Trammell 2014, 2015).
Human Footprint Types and Data Sources
The LCM was originally developed for the contiguous U.S. and was modified for use in Alaska. A complete table of direct and indirect impact scores can be found in Trammell and Aisu (2015), and additional information on methods, limitations, and other uses of the LCM in Alaska can be found in Trammell (2014, 2015). Table 1 lists the types of human footprints accounted for and the best available (at the time) datasets used to represent them statewide.
Dataset | Source | Description |
---|---|---|
Pipe Lines | ADNR | All industrial pipeline activity |
Power Lines | ADNR | All power lines for Alaska |
Telephone Lines | ADNR | All known telephone lines in Alaska |
AKEPIC | AKNHP | Invasive species database, accessed January 2015 |
BLM Trails | BLM | RS2477 trails linear features |
Ice and Rolligon Roads | BLM | Seasonal winter roads, based on permits |
Major Rivers | ADNR | Derived from the National Hydrography Dataset |
Transportation | ADNR | Includes highways, secondary roads, current and historical four-wheel drive roads, major trails (like Iditarod) as well as foot trails |
Mining | USGS | Slightly outdated estimate of current mining activity, limited to point data |
Logging | USFS | Current estimate of logging operations |
National Land Cover Database | USGS | Used for high-, medium-, and low-density urbanization, as well as agriculture |
NOTE: ADNR = Alaska Department of Natural Resources, BLM = Bureau of Land Management, AKNHP = Alaska Natural Heritage Program, USGS = U.S. Geological Survey, USFS = U.S. Forest Service. From Trammell and Aisu (2015).
We report LCM summary statistics for the whole state and for each ecoregion (as defined by Nowacki et al. 2001). The composite data layers and final LCM data are available from the Alaska Center for Conservation Science.
Alaska Scores Very High in Intactness
Statewide, there are approximately 12,500 miles (20,000 km) of major roads and over 22,900 miles (37,000 km) of trails (Figure 2). Additionally, there are approximately 5,000 square miles (1,300 square kilometers) of urban development (Trammell and Aisu 2015). In terms of modeled landscape condition, over 95% of the state is considered “very high” in intactness (LCM composite scores ≥0.80). Approximately 3% is “high” or “moderate” intactness, while less than 0.5% is classified as “very low” in intactness (Figure 2).
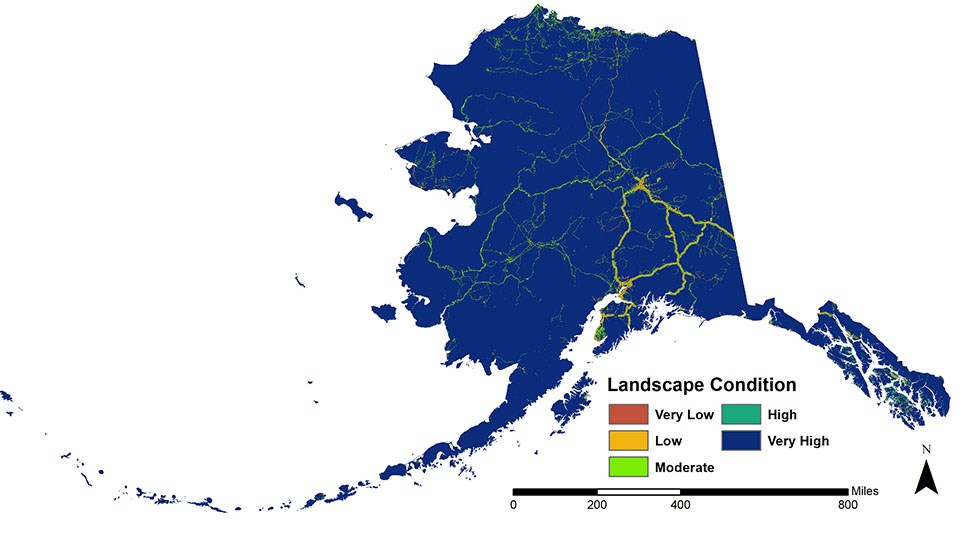
When assessed at the ecoregion scale, all ecoregions have some evidence of physical human disturbance (Figures 2 and 3). However, even the most impacted ecoregion (Cook Inlet Basin) still has a mean LCM score of 0.86, classified as “very high” intactness (Figures 2 and 3).

(The upper bar is limited to not extend beyond the maximum feasible score of 1.0.) All ecoregions had cells with values of 0.05 (highly modified) and some with values of 1.0 (no human modification).
Not surprisingly, the large majority of Alaska’s landscapes are very highly intact (Trammell and Aisu 2015) as they have not been directly modified as measured by the selected indicators and data sources (Table 1). This limited human footprint means most of the state has landscape condition values far in excess of even the most undeveloped regions of the lower-48 states (see Hak and Comer 2017). These landscape conditions are the fundamental stage supporting the migratory behavior of many of Alaska’s species.
Clearly, the landscape model that best describes intactness in Alaska is “reverse” of the model appropriate for most of the contiguous United States and the world. Alaska is best described by the reverse matrix or conservation matrix model where the human footprint occurs in patches within a background of intact and functioning ecosystems, rather than as patches of habitat with interconnecting corridors within a matrix of fragmented and modified landscapes (Schmiegelow et al. 2006).
All Alaska ecoregions exhibit some human footprint—there are no pristine ecoregions—and some ecoregions have relatively high development and, thus, low intactness (e.g., Cook Inlet and Copper River Basin; Figure 3). Yet, even the most developed regions of the state are well described by the reverse matrix model and surrounded by highly intact landscapes providing an opportunity to ensure maintenance of important migration corridors and other ecosystem services to nearby communities (Trammell and Aisu 2015).
These intact, permeable landscapes support the seasonal migration and wide-ranging behaviors of many important terrestrial species. Though not addressed here, Alaska’s very limited human modification also underpins the connectivity of the state’s freshwater systems, which support the seasonal migration and movement patterns of many important aquatic species (e.g., anadromous fish).
Although Intact, the Changing Climate Threatens Alaska’s Ecological Integrity
In interpreting these intactness values, it is important to distinguish between the concepts of landscape integrity, as measured by intactness, and ecological integrity. Landscape integrity is a function of the structure of the landscape; the intactness measure employed here specifically focuses on physical modification of the land surface by humans (or the lack thereof). Structure is just one component of ecological integrity, along with composition and function (Dale and Beyeler 2001, Tierney et al. 2009) and, in some conceptions, ecological processes (Wurtzebach and Schultz 2016). High intactness directly implies high retention of ecological structure. The very high levels of intactness found in Alaska are commonly interpreted as also implying high retention of ecological composition, function, and process. Thus, high intactness is often interpreted as equivalent to high ecological integrity (Theobald 2013).
The equivalence breaks down and can become misleading when other drivers of landscape-scale change, such as climate change impacts, become prominent (IUCN 2017). Specifically, the assumption that “intactness implies ecological integrity” is breaking down in Alaska where, arguably, the dominant driver of ecosystem change is not direct human modification of the landscape, but instead the rapidly changing climate (Chapin, III et al. 2014).
While a statewide focus masks regional differences, Alaska’s statewide annual climate warmed twice as rapidly as the contiguous U.S. in the last 60 years (ibid). The statewide average winter temperature (December, January, February) increased from 1949 to 2012 by over 6°F (3.7°C; Bieniek et al. 2014), with all of the state’s regional climate divisions exhibiting average increases of at least 3°F (1.7°C) except the Aleutians, which increased relatively little (the state’s 13 climate divisions are defined by Bieniek et al. 2012). These warming trends are projected to continue (Chapin, III et al. 2014), with winter extreme temperatures expected to continue warming much faster than other climate extremes (such as summer maximum temperatures; Lader et al. 2017). In conjunction with the greatly increased precipitation expected throughout Alaska, freezing temperatures and frozen precipitation are expected to be “… increasingly less frequent by late century” (Lader et al. 2017:2407).
Among other important observed and projected impacts of changes in climate are:
- shorter snow season and river and lake freeze-up periods, which impact seasonal migration of birds and other animals, and the northerly range expansions of species (Chapin, III et al. 2014);
- longer and drier growing seasons, with implications for shifts in major vegetation community composition and distribution, and more large fires (ibid); and
- rapidly warming (and thawing) permafrost (>56% of Alaska is underlain by permafrost vulnerable to subsidence upon thawing due to the magnitude of its ice content; ibid), leading to potentially major restructuring of landscapes due to thermokarst, subsidence, and related landform processes, including relatively rapid changes in lake and wetland spatial and size distributions (ibid).
Even in the absence of human development pressures, these climate-driven changes are impacting and will continue to impact ecosystem structure, function, and composition (ecological integrity) while not affecting intactness as typically measured. Among other effects, these changes are likely to modify landscape resistance, and therefore permeability, for many species and, ultimately, habitat suitability, undermining the interpretation of intactness as a surrogate for permeability. This highlights the need to be cautious in over-intepreting what can be inferred from high levels of intactness, and to develop measures of habitat suitability and permeability that account for more than just direct (and permanent) anthropogenic modifications of the landscape; for example, changes in freeze/thaw cycles, habitats shifts, and landform in part due to permafrost loss.
So while Alaska is, and will likely remain, very highly intact (as commonly measured), this provides limited understanding of variables likely to change landform processes that, in turn, are likely to increase landscape resistance and the state’s future capacity to support migratory and wide-ranging behaviors of Alaskan species, let alone the state’s future ecological integrity. Understanding the rate and scale of climate impacts on migration and species movements may be provided by assessing climate-connectivity potential that accounts for both landscape structure and projected rates and magnitudes of climate change (e.g., McGuire et al. 2016). While meaningful, having been developed in patch-corridor-matrix landscapes, these ideas have to be modified for relevance to the “reverse matrix”’ landscapes of Alaska and other northern latitude systems (e.g., Barber et al. 2015). Developing tools for these landscapes, and the impacts on them of changing climate, is a focus of a number of active discussions among land management agencies (e.g., National Park Service, Bureau of Land Management, U.S. Fish and Wildlife Service), boundary organizations (e.g., the Landscape Conservation Cooperatives), non-governmental organizations, and university partners in Alaska and Canada.
The future of migration in Alaska depends on the capability of landscapes to support it. While we are still working to characterize the cumulative changes expected in the region’s physical, ecological, and human landscape, it is clear current landscape permeability and ecosystem integrity will change. It is also clear, however, that intactness of habitats in Alaska provides an enviable foundation for ensuring successful adaptation to those changes. It is apt that such systems are being referred to as “landscapes of opportunity” (F. Schmiegelow, pers. comm., August 19, 2017).
Fore More Information
See the Alaska Department of Fish and Game’s Crucial Habitat Assessment Tool (CHAT), which uses the LCM.
For additional uses of and description of the LCM in Alaska, see the Bureau of Land Management Rapid Ecoregional Assessments (REAs).
For additional information on the upcoming session at the North American Congress for Conservation Biology discussing Landscapes of Opportunity: Conserving Large Functioning Systems in Northern Canada and Alaska.
REFERENCES
Barber, Q., S. Nielsen, and A. Hamann. 2016.
Assessing the vulnerability of rare plants using climate change velocity, habitat connectivity, and dispersal ability: a case study in Alberta, Canada. Regional Environmental Change 16(5), 1433-1441.
Belote, R. T., M. S. Dietz, C. N. Jenkins, P. S. McKinley, G. H. Irwin, T. J. Fullman, J. C. Leppi, and G. H. Aplet. 2017.
Wild, connected, and diverse: building a more resilient system of protected areas. Ecological Applications 27(4):1050-1056. DOI: 10.1002/eap.1527
Bieniek, P. A., J. E. Walsh, R. L. Thoman, and U. S. Bhatt. 2014.
Using climate divisions to analyze variations and trends in Alaska temperature and precipitation. Journal of Climate 27: 2800-2818. DOI: 10.1175/JCLI-D-13-00342.1.1
Bieniek, P. A., U. S. Bhatt, R. L. Thoman, H. Angeloff, J. Partain, J. Papineau, F. Fritsch, E. Holloway, J. E. Walsh, C. Daly, M. Shulski, G. Hufford, D. F. Hill, S. Calos, R. Gens. 2012.
Climate divisions for Alaska based on objective methods. Journal of Applied Meteorology and Climatology 51: 1276-1289. DOI: 10.1175/JAMC-D-11-0168.1.
Carter, S. K., N. B. Carr, K. H. Miller, and D. J. A. Wood, eds. 2017.
Multiscale guidance and tools for implementing a landscape approach to resource management in the Bureau of Land Management. U.S. Geological Survey Open-File Report 2016–1207, 79 p.
Chapin, F. S., III, S. F. Trainor, P. Cochran, H. Huntington, C. Markon, M. McCammon, A. D. McGuire, and M. Serreze. 2014.
Chapter 22: Alaska. In: Melillo, J. M., T. C. Richmond, and G. W. Yohe, eds, Climate Change Impacts in the United States: The Third National Climate Assessment. U.S. Global Change Research Program, pgs 514-536. doi:10.7930/J00Z7150.
Comer, P. J. and J. Hak. 2012.
Landscape Condition in the Conterminous United States. Spatial Model Summary. NatureServe, Boulder, CO. (accessed 19 Feb 2018)
Dale, V. H. and S. C. Beyeler. 2001.
Challenges in the development and use of ecological indicators. Ecological Indicators 1: 3-10.
Forman, Richard T. T. 1995.
Land Mosaics: The ecology of landscapes and regions. Cambridge University Press, Cambridge, U.K., 632p.
Hak, J. C. and P. J. Comer. 2017.
Modeling landscape condition for biodiversity assessment - Application in temperate North America. Ecological Indicators 82: 206-216.
International Union for Conservation of Nature and Natural Resources (IUCN). 2017.
Threats Classification Scheme (Version 3.2). (accessed February 16, 2018).
Lader, R., J. E. Walsh, U. S. Bhatt, and P. Bieniek. 2017.
Projections of Twenty-First-Century climate extremes for Alaska via dynamical downscaling and quantile mapping. Journal of Applied Meteorology and Climatology 56: 2393-2409. DOI: 10.1175/JAMC-D-16-0415.1.
McGuire, J. L., J. J. Lawler, B. H. McRae, T. A. Nuñez, and D. M. Theobald. 2016.
Achieving climate connectivity in a fragmented landscape. Proceedings of the National Academy of Science 113(26): 7195-7200.
Nowacki, G. J., P. Spencer, M. Fleming, T. Brock, and T. Jorgenson. 2001.
Ecoregions of Alaska: 2001. U.S. Geological Survey Open-File Report 02-297 (map). (accessed 9 Apr 2018)
Schmiegelow, F. K. A., S. G. Cumming, S. Harrison, S. Leroux, K. Lisgo, R. Noss, and B. Olsen, 2006.
Conservation beyond crisis management: A reverse-matrix model. A discussion paper for the Canadian BEACONs Project: vol 1, pgs 1-7. (accessed 12 Feb 2018)
Singleton, P. H., W. L. Gaines, J. F. Lehmkuhl. 2002.
Landscape permeability for large carnivores in Washington: a geographic information system weighted-distance and least-cost corridor assessment. Res. Pap. PNW-RP-549. Portland, OR: U.S. Department of Agriculture, Forest Service, Pacific Northwest Research Station, 89 p. (accessed 5 Feb 2018)
Theobald, D. M. 2013.
A general model to quantify ecological integrity for landscape assessments and US application. Landscape Ecology 28(7):1859-1874. doi: 10.1007/s10980-013-9941-6.
Tierney, G. L., D. Faber-Langendoen, B. R. Mitchell, W. G. Shriver, and J. P. Gibbs. 2009.
Monitoring and evaluating the ecological integrity of forest ecosystems. Frontiers in Ecology and the Environment 7(6): 308-316.
Trammell, E. J. 2015.
Landscape and Ecological Integrity. In: Trammell, E.J., M. L. Carlson, N. Fresco, T. Gotthardt, M. L. McTeague, and D. Vadapalli. Eds., North Slope Rapid Ecoregional Assessment. Prepared for the Bureau of Land Management, U.S. Department of the Interior. University of Alaska, Anchorage, Alaska.
Trammell, E. J. 2014.
Landscape and Ecological Integrity. In: Trammell, E. J., M. L. McTeague, and M. L. Carlson, eds, Yukon River Lowlands – Kuskokwim Mountains – Lime Hills Rapid Ecoregional Assessment Technical Supplement. Prepared for the U.S. Department of the Interior, Bureau of Land Management, Denver, Colorado.
Trammell, E. J. and M. Aisu. 2015.
Development of a landscape integrity dataset for the Alaska Crucial Habitat Assessment Tool. Prepared for the Alaska Department of Fish and Game. University of Alaska, Anchorage. (accessed 12 Feb 2018)
Tucker, M. A. and 114 co-authors. 2018.
Moving in the Anthropocene: global reductions in terrestrial mammalian movements. Science 359: 466-469.
Wurtzebach, Z. and C. Schultz. 2016.
Measuring ecological integrity: history, practical applications, and research opportunities. Bioscience 66: 446-457. doi: 10.1093/biosci/biw037.
Last updated: February 7, 2019