Part of a series of articles titled Alaska Park Science - Volume 16 Issue: Science in Alaska's Arctic Parks.
Article
Small Mammals as Indicators of Climate, Biodiversity, and Ecosystem Change
By Andrew G. Hope, Kansas State University; Eric Waltari, Aaron Diamond AIDS Research Center; Nathan R. Morse, Kansas State University; Joseph A. Cook, University of New Mexico; Melanie J. Flamme, National Park Service; Sandra L. Talbot, U.S. Geological Survey
Climate is a driving evolutionary force for biodiversity in high-latitude Alaska. This region is complex and dynamic with high annual variation in temperature and light. Through deeper time, Alaska has experienced major climate extremes over much longer periodicity. For example, the Quaternary Period (the last ~2.5 million years), commonly known as the Ice Age, was punctuated by more than 20 major glacial-interglacial cycles.
Climate is a driving evolutionary force for biodiversity in high-latitude Alaska. This region is complex and dynamic with high annual variation in temperature and light. Through deeper time, Alaska has experienced major climate extremes over much longer periodicity. For example, the Quaternary Period (the last ~2.5 million years), commonly known as the Ice Age, was punctuated by more than 20 major glacial-interglacial cycles.
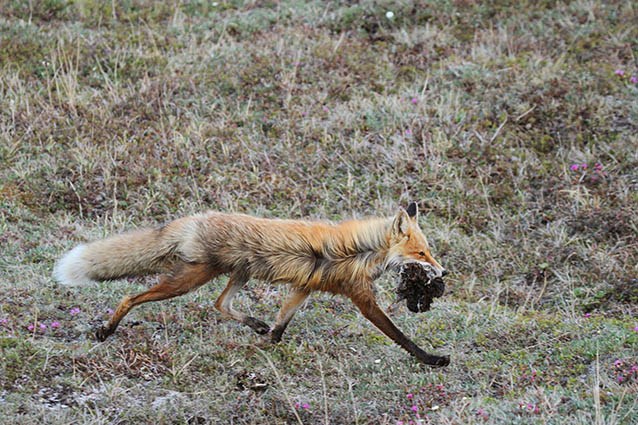
Courtesy Jared Hughey
Each extended glacial phase periodically transitioned into a shorter interglacial warm phase. These climate reversals melted continental ice sheets to expose corridors for reinvasion of terrestrial species, particularly those associated with forested habitats further south. Those species that survived at northern latitudes through repeated glacial-interglacial cycles formed the Arctic tundra communities that persist today.
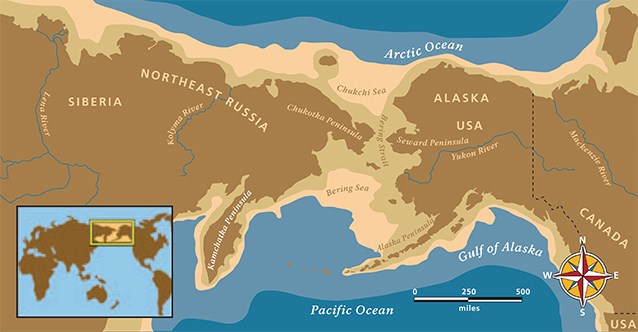
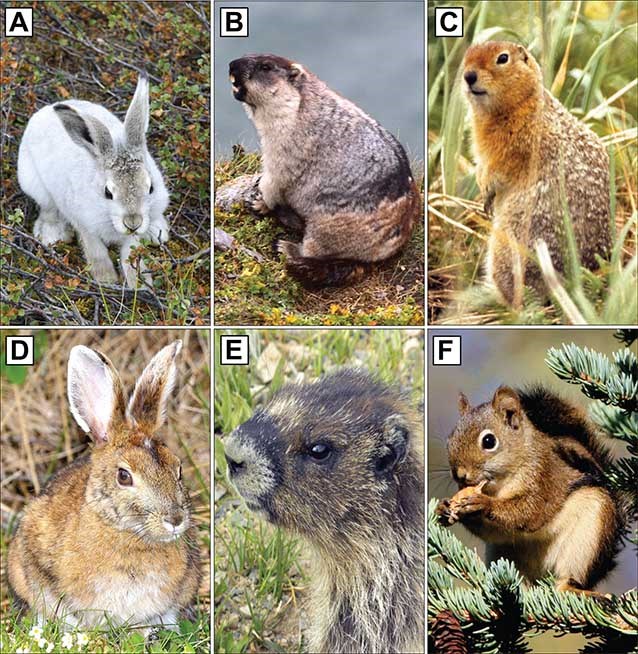
Creative Commons (creativecommons.org)
Methods to Understand Changing Ecosystems
One of the central challenges for the National Park Service (NPS) is to understand how natural resources (particularly biodiversity) are responding to change through time (Marcot et al. 2015). Understanding how species have responded to past episodes of environmental change provides comparative knowledge for current changes and predictive ability to envision future trends. One way we can access evidence from different temporal scales is by combining knowledge from multiple sources.
For instance, we can compare fossil evidence of the extent of ancient distributions with present species’ ranges, often suggesting dramatic distributional shifts through time. Field specimen collections spanning multiple decades (Cook et al. 2004), provide valuable samples through time for DNA or isotopic analyses that can highlight changes in regional diversity. Evolutionary histories of species leave predictable signatures within DNA sequences that can indicate how species have responded to changing environments, through movement, population size changes, adaptation, or often through complex interactions between species. At the most recent temporal scale, ecological modeling and analyses can assess recent changes in populations as they react to current climate.
Monitoring Small Mammals
Small mammals are a valuable group of wildlife for understanding fine-scale responses to environmental change. Twenty-three years of sampling in Denali National Park and Preserve has shown that long-term ecological monitoring provides critical information on population and community changes (MacCluskie and Oakley 2005). Small mammals in Alaska are diverse for high-latitudes, reflecting evolutionary origins from both Eurasia, further south in North America, and including unique populations that are a consequence of local adaptation over multiple glacial phases. Small mammals are also intermediate trophic components of communities where they rely on vegetation, seeds, and arthropods for food, and interact with higher vertebrates through competition for resources or as a source of prey (Krebs et al. 2014).
They have rapid generation times and are often found at exceptionally high abundances, making them relatively easy to locate, capture, and process from a wild setting, particularly considering the many logistic challenges associated with field access and inventory through Alaska. Unlike most birds that migrate seasonally to compensate for fluctuating climate and food resources, small mammals are resident and most do not hibernate, meaning that they respond and adapt to year-round conditions.

An Integrated Analytical Framework for Community Assessments
A key advantage of monitoring small mammals is the “library” of accumulated specimens archived in museums and associated ecological data resulting from decades of standardized field sampling and analysis. As a consequence of robust field efforts (Cook et al. 2004, 2005), knowledge of the evolutionary and natural history of small mammals in Alaska is relatively comprehensive. We can now begin to interpret how whole communities change through time for a given region.
Analysis of accumulated data is facilitated by new statistical and genetic methods not possible only a decade ago (Hope et al. 2013). To demonstrate, we gathered genetic sequences and locality information from museum databases for over 25 species of small mammals occurring within Alaska, including tundra and boreal forest species, and representing shrews, voles, squirrels, rabbits, and small carnivores. Our results indicate that species associated with forests in Alaska arrived here together through rapid range expansion since the end of the last glacial phase as climate warmed (Hope et al. 2015). Many are still experiencing population growth and continued range expansion. Tundra species, however, exhibited more idiosyncratic responses, whereas some species recently expanded, others exhibited stable or declining populations.
In addition to interpreting genetic information from museum specimens, we performed ecological analyses of climate data associated with the current distribution of each species. Climate tolerances of species (e.g. temperature or rainfall extremes) for the present time are often a strong predictor of where they occur. We used these climatic “envelopes” to verify the genetic and fossil evidence and better understand how distributions have changed through time, and how they may be expected to change into the future. Future predictions indicate the possible trend (increase or decrease in population size), magnitude, and physical location of species ranges into the next several decades. Finally, by overlaying predictions from multiple species, we mapped changes in small mammal diversity through time, independently for both tundra and forest communities (Figure 3) and with all study species combined (Figure 4).
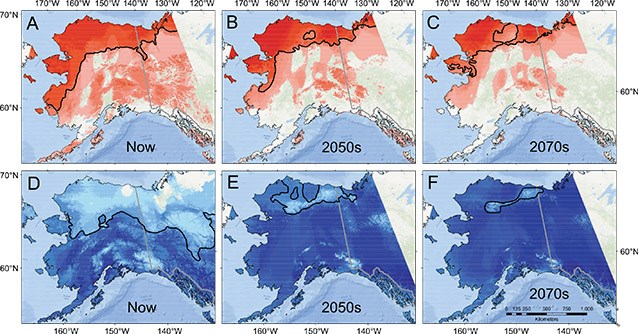
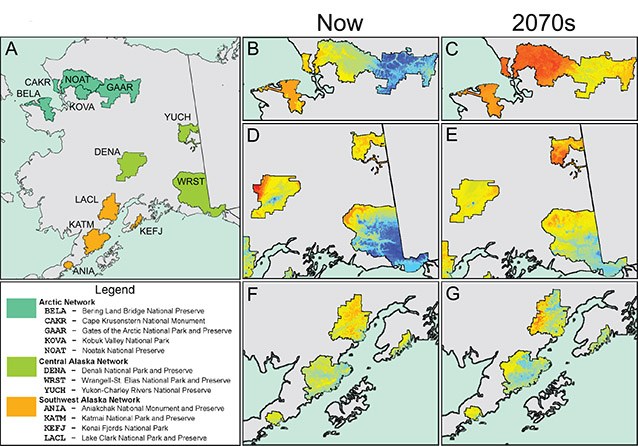
Trends in Diversity and Community Turnover
Predicted changes for total small mammal diversity into the next century vary across Alaska parks (Figure 4). In the Arctic, mammal diversity exhibits a strong longitudinal gradient with more species predicted in the west. Future predictions indicate an overall increase in diversity, although the distribution of diversity will become more even (Figure 5). Increasing diversity is generally considered beneficial; however, this increase is largely due to the rapid northward movement of forest species, whereas tundra species are contracting their range more slowly. Different velocities of change among forest and tundra species (Figure 3) will broaden overlap between these communities through time and increase the complexity of interactions among them. In the central forested region, the total number of species is not predicted to change, although the distribution of this diversity will change as species are predicted to invade higher elevations and so increasing total occupancy in this region.
Within Southwest Alaska, the number of species is predicted to decrease slightly as total diversity shifts along both latitudinal and elevational gradients. It is possible that other species currently occurring further south, such as Keen’s deer mouse, may shift north and west, maintaining or increasing the total small mammal diversity through this region. Recent studies have also suggested that climate changes may reduce the extent of boreal forests further south in Alaska, giving way to other novel habitats such as deciduous forest or grassland (e.g., Wolken et al. 2011), and by extension, changes in the associated mammal communities.
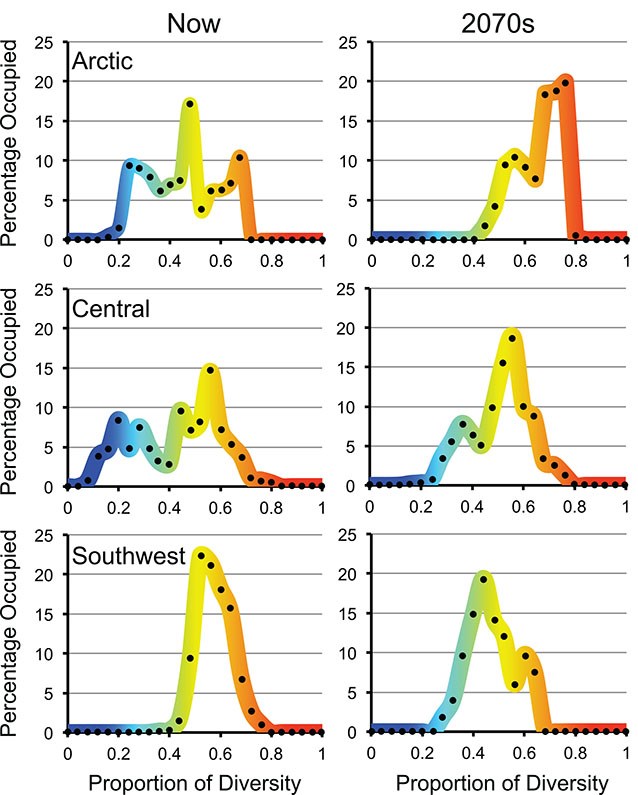
Conclusion
This is a time of rapid environmental changes in Alaska and effective management of natural resources benefits from detailed knowledge of the past and present, and our most informed predictions of future biotic responses to these changes (Barnosky et al. 2012). Species that have evolved within tundra habitats over multiple glacial cycles are not only best adapted to high-latitude and high-elevation environments, but may also respond more slowly to change. This equates to a broadening overlap between distinct communities as forest species advance along environmental gradients faster than tundra species retreat.
Less certain is how different communities will interact in regions of overlap, so-called contact zones, such as occurs across latitudinal, longitudinal, and elevational gradients through many Alaska parks. High-latitude and high-elevation areas may constitute future refugial areas for tundra and alpine species respectively and these regions within Alaska parks will likely constitute critical areas for future research that informs resource management. Studies of hybridization, competition, disease, associated species such as parasites, physiological tolerances, phenology, and evolutionary adaptation to novel environments could all be facilitated by focusing on small mammal communities and continuing to build spatially extensive and temporally deep archives from parks.
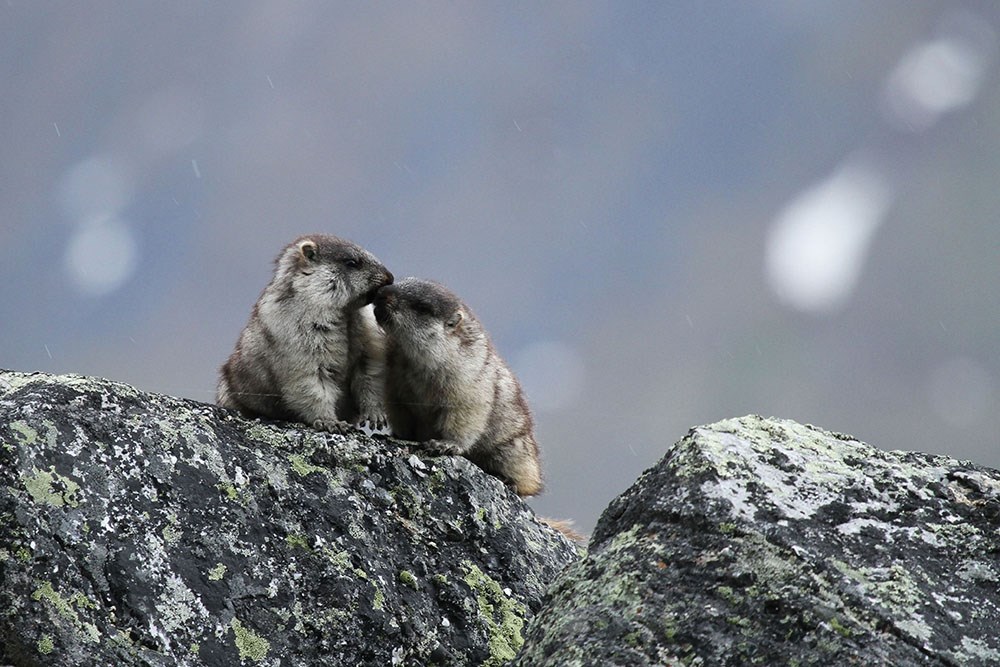
Courtesy Jared Hughey
References
Barnosky, A. D., E. A. Hadly, J. Bascompte, E. L. Berlow, J. H. Brown, M. Fortelius, W. M. Getz, J. Harte, A. Hastings, P. A. Marquet, N. D. Martinez, A. Mooers, P. Roopnarine, G. Vermeij, J. W. Williams, R. Gillespie, J. Kitzes, C. Marshall, N. Matzke, D. P. Mindell, E. Revilla, and A. B. Smith. 2012.
Approaching a state shift in Earth’s biosphere. Nature 486:52-58.
Cook, J., N. Dawson, S. MacDonald, and A. Runck. 2004.
Mammal diversity: Inventories of Alaska National Parks stimulate new perspectives. Alaska Park Science 3(2):22-27.
Cook, J. A, E. P. Hoberg, A. Koehler, H. Henttonen, L. Wickström, V. Haukisalmi, K. Galbreath, F. Chernyavski, N. Dokuchaev, A. Lahzuhtkin, S. O. MacDonald, A. Hope, E. Waltari, A. Runck, A. Veitch, R. Popko, E. Jenkins, S. Kutz, and R. Eckerlin. 2005.
Beringia: intercontinental exchange and diversification of high latitude mammals and their parasites during the Pliocene and Quaternary. Mammal Study 30:S33-S44.
Hope, A. G., E. Waltari, J. L. Malaney, D. C. Payer, J. A. Cook, and S. L. Talbot. 2015.
Arctic biodiversity: increasing richness accompanies shrinking refugia for a cold-associated tundra fauna. Ecosphere 6:article 159.
Hope, A. G., E. Waltari, D. C. Payer, J. A. Cook, and S. L. Talbot. 2013.
Future distribution of tundra refugia in northern Alaska. Nature Climate Change 3:931-938.
Krebs, C. J., R. Boonstra, S. Boutin, A.R.E. Sinclair, J. N. M. Smith, B. S. Gilbert, K. Martin, M. O’Donoghue, and R. Turkington. 2014.
Trophic dynamics of the boreal forests of the Kluane region. Arctic 67:71-81.
MacCluskie M. and K. Oakley. 2005.
Central Alaska Network Vital Signs Monitoring Plan Phase III Report. National Park Service, Fairbanks, Alaska.
Marcot, B. G., M. T. Jorgenson, J. P. Lawler, C. M. Handel, and A. R. DeGange. 2015.
Projected changes in wildlife habitats in Arctic natural areas of northwest Alaska. Climatic Change 130:145-154.
Wolken, J. M., T. N. Hollingsworth, T. S. Rupp, F. S. Chapin III, S. F. Trainor, T. M. Barrett, P. F. Sullivan, A. D. McGuire, E. S. Euskirchen, P. E. Hennon, E. A. Beever, J. S. Conn, L. K. Crone, D. V. D’Amore, N. Fresco, T. A. Hanley, K. Kielland, J. J. Kruse, T. Patterson, E. A. G. Schuur, D. L. Verbyla, and J. Yarie. 2011.
Evidence and implications of recent and projected climate change in Alaska’s forest ecosystems. Ecosphere 2:article 124.
Tags
- aniakchak national monument & preserve
- bering land bridge national preserve
- cape krusenstern national monument
- denali national park & preserve
- gates of the arctic national park & preserve
- katmai national park & preserve
- kenai fjords national park
- kobuk valley national park
- lake clark national park & preserve
- noatak national preserve
- wrangell - st elias national park & preserve
- arctic
- science
- wildlife
- small
- mammals
- climate change
Last updated: March 6, 2017