Last updated: November 26, 2018
Article
Geological monitoring of caves and associated landscapes
Rickard S. Toomey III
Mammoth Cave International Center for Science and Learning, Western Kentucky University, 1906 College Heights Blvd. #31066, Bowling Green, Kentucky 42101-1066, USA
Toomey, R.S., III, 2009, Geological monitoring of caves and associated landscapes, in Young, R., and Norby, L., Geological Monitoring: Boulder, Colorado, Geological Society of America, p. 27–46, doi: 10.1130/2009.monitoring(02). For permission to copy, contact editing@geosociety.org. ©2009 The Geological Society of America. All rights reserved.
INTRODUCTION
Caves are naturally occurring underground voids. They occur in many types of rocks in many ecosystems. Common types of caves include solutional (karst) caves, lava tubes, sea caves, talus caves, regolith caves (formed by soil piping) and glacier caves (ice-walled caves). Caves range in size from only a few meters long to over 100 km long; the longest known cave, Mammoth Cave in Kentucky, is 580 km long. They also vary considerably in complexity, depth, volume, number of entrances, and many other fundamental parameters.
A concept closely related to solutional caves is karst. Karst is a landscape that forms through the dissolution of soluble rock. The most common soluble rock types are limestone and dolomite. Other types of soluble rock that form karst include marble, various evaporates (e.g., gypsum, anhydrite, and halite), and occasionally sandstone or quartzite. Karst landscapes are characterized by internal drainage, losing streams, sinkholes, caves, and springs. Karst is a prevalent landscape; ~20% of the United States is karst. Veni et al. (2001) provides a good introduction to karst. Books such as Moore and Sullivan (1997) and Gillieson (1996) provide somewhat more detailed, but still easily understood
discussions of the subject.
Caves are significant, non-renewable, geological resources. They are significant in their own right and also because they house other signifi cant resources including geological resources (mineral deposits, paleontological remains, etc), biological resources (cave adapted and/or dependent microbiology, invertebrates, and vertebrates), and cultural resources (archaeological,
historical, religious, and cultural materials, remains, and values). Jones et al. (2003) provides a good introduction to some of the aspects of managing these cave resources. Monitoring of the fundamental vital signs of caves is important in protecting the caves as a whole and the resources contained in them. Hamilton-Smith (2002) discusses the rationale and context for cave monitoring. His discussion includes an introduction to different intellectual models upon which existing monitoring strategies are based. Although its focus is on conservation and restoration, rather than on monitoring, Hildreth-Werker and Werker (2006) provides a strong introduction to many cave resources and types of human impacts that may affect them.
Caves and karst are a very important geological resource in the U.S. National Park system. At least 3900 caves have been identified in 81 national parks. Karst, but no recorded caves, occurs in an additional 39 parks.
Monitoring of geological resources and processes in caves is difficult to separate from two related activities. The first of these other activities is inventory. The second is recurring, long-term scientific research. It can often be difficult to distinguish these three activities. Often there may be overlap among them, and a project that starts as either an inventory or recurring scientific study may become a monitoring project.
Inventory is a crucial prerequisite and companion to monitoring. Inventory is necessary to determine what vital signs need to be monitored, to choose appropriate places to monitor, to determine what monitoring techniques are most appropriate, and to establish the initial conditions from which the monitoring will take place. DuChene (2006) provides a good introduction to cave inventory, including a discussion of both rationale and techniques. Many of the techniques used in monitoring are also used in inventory. For example, the same instruments that are used to measure cave climate, water quality, or visitor impacts are used to make an inventory of the same parameters. Inventory differs from monitoring in that inventory determines only the initial state of a vital sign, cave, or cave resource.
Recurring, long-term scientific research is more difficult to distinguish from monitoring as discussed in this volume. An example of recurring scientific research might be a study of dissolution rates of limestone in a cave passage. Such a study might involve taking measurements of a parameter (such as weight of a limestone plate or the retreat of a wall from a set point) at regular intervals using a set protocol. In this way the activity would be the same as monitoring. However, for the purposes of this manual, monitoring should have the potential to influence management action. That is, it should be measuring a parameter that is likely to be affected by threats to vulnerable resources or possible management actions, and which will show a change if management actions are altered. In the example above, it is unlikely that most management actions will affect limestone dissolution rates; instead, it would mainly be of interest for its purely scientific value. For this reason the dissolution study might not be considered monitoring in this manual. The difference between monitoring, as used in this manual, and recurring scientific research is both tenuous and fluid. As we learn more about human impacts on cave and surface systems, many things that may start as recurring scientific research may be found to provide important information about impacts that had been previously unforeseen. In addition, although some impacts cannot be directly affected by the actions of resource managers on a park (or even regional) scale, monitoring may provide important information with which to evaluate, illustrate, or address broader national or multinational impacts. Global warming provides a good example. Monitoring cave temperatures may provide important information on patterns of warming in different areas. Such monitoring will thus serve an important purpose, even if the cave managers cannot take action that will reverse the warming.
A study that starts as a recurring, long-term scientific study may become a monitoring project, as the understanding of human impacts, potential management actions, and the processes being studied changes. Monitoring projects may also contribute data that can be used for scientific studies that seek to understand cave processes. Indeed, as one implements a monitoring project, it is important to try to set it up in a way that provides as much generally useful data as possible.
The Carlsbad Caverns National Park Cave and Karst Management Plan Environmental Assessment contains a brief, but well-developed cave monitoring plan (Carlsbad Caverns National Park, 2006). It discusses the existing monitoring at the park, the rationale that will be used to decide whether new or additional monitoring should take place, and the procedures to be followed in developing new monitoring projects. It provides excellent guidance on how to develop cave monitoring projects.
An important issue in the development of this chapter is that caves are found at the intersection of the geological, hydrological, biological, and atmospheric disciplines. Many of their resources and processes cannot be readily separated into simple categories. For example, evidence of microbiological roles in some speleogenesis (cave formation) and mineral deposition continues to mount (Barton and Luiszer, 2005; Barton et al., 2001; Northup and Lavoie, 2001). Are these microbiological processes best considered biological, geological, both, neither, or something in between? Cave climate, although probably best considered as an air resources issue, has very profound effects on many aspects of caves and cave resources. In fact, it may be the single most important aspect of many caves to monitor for resource protection. In general, I have attempted to discuss the main vital signs that can provide important information for managing caves as a whole or important geological resources within them. In the case of obviously hydrological vital signs, I have generally discussed them with less detail. Numerous agencies, including the National Park Service (NPS) Water Resources Division, U.S. Geological Survey Water Resources Program, the Environmental Protection Agency Office of Water, and various state agencies, can provide additional guidance on water monitoring, as well as access to hydrologists to assist in developing monitoring programs.
A related issue with cave monitoring is that caves contain a range of “geological settings,” some of which overlap with traditional surface geological settings that are discussed elsewhere in this book. Managers may find other chapters assist in developing monitoring programs for caves and cave resources. For example, a cave may have active streams or lakes that could be analyzed and monitored in ways at least similar to surface streams or lakes. Lava tubes are obviously volcanic features; some volcanic monitoring techniques may be applicable to at least the youngest lava tubes, such as those found on the island of Hawai’i. Caves may contain very significant paleontological resources; monitoring of these resources will assist in protecting them, but may also provide more general information about impacts to the individual caves.
VITAL SIGN: CAVE METEOROLOGY
Cave meteorology refers to the microclimate and air composition of the cave. It includes parameters such as air temperature, water vapor content of air (variously measured and expressed as absolute humidity, relative humidity, saturation debt, or dew point), evaporation rates, condensation, air movement (speed, direction, flux, etc.), barometric pressure, carbon dioxide level, radon level, and hazardous gas levels (methane, hydrogen sulfide, carbon monoxide). In addition to these air parameters, cave sediment temperature, water temperature, and rock temperature are commonly monitored with cave meteorology.
Cave meteorological parameters are among the most commonly monitored parameters in cave management. There are three basic reasons for this fact. First, many cave processes are very sensitive to changes in cave meteorological parameters. Microclimate can influence the form and morphology of the mineralogy and the crystal structure of speleothems (secondary mineralogical deposits that form in caves, e.g., stalactites and stalagmites) (Hill and Forti, 1997). Decreases in humidity can lead to a decline in cave-adapted animal life. For example, an increase in cave temperature may turn an ideal bat hibernaculum into a cave that is not used by bats. Increases in the concentration of carbon dioxide in cave air can lead to dissolution of cave formations. Paleontological, archaeological and cultural resources in a cave can be damaged by changes in cave microclimate. Second, cave meteorology is relatively sensitive to human activities in caves. The presence of people in a cave can significantly alter the microclimate of some low-energy flow caves, and large-scale tourist activities will alter the microclimate even in high-energy flow caves. Third, some of the cave meteorological parameters, such as temperature and evaporation, are among the most readily monitored cave parameters (at least at a very basic level).
It is difficult to generalize about the variability of cave meteorological parameters, both because of the difference in caves and because different parameters may show different patterns. In general, the variability of temperature, humidity, evaporation, condensation, and carbon dioxide levels is greater near the entrances to a cave. Deep in the cave these parameters may vary by only very small amounts (tenths of a degree in the case of air temperature, or fractions of a percent in the case of relative humidity). Sediment, water, and rock temperatures tend to be much less variable than air temperatures in the same area. Air flow (wind speed and direction) is much more difficult to generalize. Near entrances, significant airflow may be expected; however, in constricted areas deep in the cave, air flow may also be highly variable. In many cases, cave meteorological readings are best interpreted with surface meteorological readings from the same area.
Monitoring of cave microclimate may be needed for several different reasons. Because cave climate conditions are both broadly sensitive to and dependent on external climate conditions, monitoring cave climate conditions (especially in caves that are rarely visited) can provide information on natural regional climate change. More importantly, from a management perspective, monitoring helps to assess risk to resources due to cave climate changes (natural or artificial). In some caves, the presence of, amounts of, and variations in exotic gases (such as H2S, CO, CH4, etc.) are important to understanding the cave processes and to protecting people who may enter such caves. Because of the sensitivity of many cave resources to changes in cave microclimate, monitoring should take place when there are significant resources that could be impacted by changes in cave climate, when management actions might result in a change in climate in a cave, or to provide control data for a nearby cave undergoing a management
action.
When developing a cave meteorology monitoring program, it is important to consider both the range of values that can be anticipated for various parameters, and how much change in a variable will be significant in affecting cave processes, or likely to result from human impacts or management actions. This type of information would be obtained through an inventory process where a variety of parameters may be measured using either spot measurements or relatively short runs with data logging instruments. This type of inventory will help establish how the parameters vary in the cave over time. It will also provide important data that allows selection of the most appropriate instruments for measuring various parameters.
Radon concentration in cave air is an important parameter that is monitored in a number of caves (including many NPS show caves). Radon variation data can be used to examine cave airflow patterns (i.e., Pflitsch and Piasecki, 2003). However, most, if not all, radon monitoring is driven by regulatory requirements aimed at safeguarding human health. The NPS Air Resources Division is currently revising radon monitoring procedures for NPS caves (B. Carson, Mammoth Cave National Park, personal commun., 2008). In addition, the National Caves Association (the primary show caves industry group in the United States) has developed industry standards for monitoring and mitigating radon exposures in show caves (Aley et al., 2006).
Methods
Methods of monitoring cave meteorology vary from the extremely cheap and easy to the very expensive and complex. The main variable in cost is frequently the instruments used to measure and record the data. The more expensive instruments often provide greater accuracy and precision, greater storage
capacity and greater flexibility in timing of measurements. Cigna (2002) provides good information on a range of monitoring solutions for cave meteorology. The National Park Service’s Cumberland Piedmont Inventory and Monitoring Network has developed protocols for monitoring some aspects of cave meteorology, in particular air temperature, humidity, and airflow (J. Jernigan, Mammoth Cave National Park, personal commun., 2008). Copies of these protocols are available from the Cumberland Piedmont I&M Network (http://science.nature.nps.gov/im/units/cupn/). In addition, a group of resource management specialists from the NPS are working to expand those protocols to include additional aspects of cave meteorology. This work is scheduled to be completed in 2010.
When these protocols are finished, they will provide very useful guidance for monitoring these parameters. The protocols being developed focus mainly on mixed use of handheld instrumentation and datalogged sensors, and thus would generally fall in the medium level of cost, technology, and expertise. They also focus on developing an understanding of the spatial and temporal variation in parameters as part of developing a monitoring program.
In general, much basic monitoring can be accomplished by relatively inexpensive people, such as Student Conservation Association interns (SCAs), volunteers, citizen scientists, or students with some training using handheld instruments (such as thermometers, sling psychrometers, anemometers, or gas meters; Fig. 1). The timing between measurements will vary depending on the type of issues being examined. For deep cave measurements in caves not subjected to management actions that might alter cave climate, seasonal measurements may provide adequate data. If caves are subject to management actions that might alter climate (or if they serve as controls) more frequent measurement (monthly or weekly) might be appropriate. Because of variation in instruments, calibration is frequently necessary. Many relatively inexpensive thermometers can be calibrated against either a master thermometer or known temperature points (such as an ice and water mix). Cigna (2002) recommends 0.1 °C accuracy and precision for general purpose cave monitoring. Some specialized monitoring may require more accuracy and precision.
Evaporation and condensation are important meteorological processes that may affect cave resources. Evaporation rates may affect mineral growth rates and styles, desiccation potential of cave fauna, and cave pool extents and depths. Evaporation can be measured relatively easily with simple evaporation pans with standardized amounts of water. However, in areas near entrances, it may be important to protect evaporation pans from wildlife.
A concept closely related to solutional caves is karst. Karst is a landscape that forms through the dissolution of soluble rock. The most common soluble rock types are limestone and dolomite. Other types of soluble rock that form karst include marble, various evaporates (e.g., gypsum, anhydrite, and halite), and occasionally sandstone or quartzite. Karst landscapes are characterized by internal drainage, losing streams, sinkholes, caves, and springs. Karst is a prevalent landscape; ~20% of the United States is karst. Veni et al. (2001) provides a good introduction to karst. Books such as Moore and Sullivan (1997) and Gillieson (1996) provide somewhat more detailed, but still easily understood
discussions of the subject.
Caves are significant, non-renewable, geological resources. They are significant in their own right and also because they house other signifi cant resources including geological resources (mineral deposits, paleontological remains, etc), biological resources (cave adapted and/or dependent microbiology, invertebrates, and vertebrates), and cultural resources (archaeological,
historical, religious, and cultural materials, remains, and values). Jones et al. (2003) provides a good introduction to some of the aspects of managing these cave resources. Monitoring of the fundamental vital signs of caves is important in protecting the caves as a whole and the resources contained in them. Hamilton-Smith (2002) discusses the rationale and context for cave monitoring. His discussion includes an introduction to different intellectual models upon which existing monitoring strategies are based. Although its focus is on conservation and restoration, rather than on monitoring, Hildreth-Werker and Werker (2006) provides a strong introduction to many cave resources and types of human impacts that may affect them.
Caves and karst are a very important geological resource in the U.S. National Park system. At least 3900 caves have been identified in 81 national parks. Karst, but no recorded caves, occurs in an additional 39 parks.
Monitoring of geological resources and processes in caves is difficult to separate from two related activities. The first of these other activities is inventory. The second is recurring, long-term scientific research. It can often be difficult to distinguish these three activities. Often there may be overlap among them, and a project that starts as either an inventory or recurring scientific study may become a monitoring project.
Inventory is a crucial prerequisite and companion to monitoring. Inventory is necessary to determine what vital signs need to be monitored, to choose appropriate places to monitor, to determine what monitoring techniques are most appropriate, and to establish the initial conditions from which the monitoring will take place. DuChene (2006) provides a good introduction to cave inventory, including a discussion of both rationale and techniques. Many of the techniques used in monitoring are also used in inventory. For example, the same instruments that are used to measure cave climate, water quality, or visitor impacts are used to make an inventory of the same parameters. Inventory differs from monitoring in that inventory determines only the initial state of a vital sign, cave, or cave resource.
Recurring, long-term scientific research is more difficult to distinguish from monitoring as discussed in this volume. An example of recurring scientific research might be a study of dissolution rates of limestone in a cave passage. Such a study might involve taking measurements of a parameter (such as weight of a limestone plate or the retreat of a wall from a set point) at regular intervals using a set protocol. In this way the activity would be the same as monitoring. However, for the purposes of this manual, monitoring should have the potential to influence management action. That is, it should be measuring a parameter that is likely to be affected by threats to vulnerable resources or possible management actions, and which will show a change if management actions are altered. In the example above, it is unlikely that most management actions will affect limestone dissolution rates; instead, it would mainly be of interest for its purely scientific value. For this reason the dissolution study might not be considered monitoring in this manual. The difference between monitoring, as used in this manual, and recurring scientific research is both tenuous and fluid. As we learn more about human impacts on cave and surface systems, many things that may start as recurring scientific research may be found to provide important information about impacts that had been previously unforeseen. In addition, although some impacts cannot be directly affected by the actions of resource managers on a park (or even regional) scale, monitoring may provide important information with which to evaluate, illustrate, or address broader national or multinational impacts. Global warming provides a good example. Monitoring cave temperatures may provide important information on patterns of warming in different areas. Such monitoring will thus serve an important purpose, even if the cave managers cannot take action that will reverse the warming.
A study that starts as a recurring, long-term scientific study may become a monitoring project, as the understanding of human impacts, potential management actions, and the processes being studied changes. Monitoring projects may also contribute data that can be used for scientific studies that seek to understand cave processes. Indeed, as one implements a monitoring project, it is important to try to set it up in a way that provides as much generally useful data as possible.
The Carlsbad Caverns National Park Cave and Karst Management Plan Environmental Assessment contains a brief, but well-developed cave monitoring plan (Carlsbad Caverns National Park, 2006). It discusses the existing monitoring at the park, the rationale that will be used to decide whether new or additional monitoring should take place, and the procedures to be followed in developing new monitoring projects. It provides excellent guidance on how to develop cave monitoring projects.
An important issue in the development of this chapter is that caves are found at the intersection of the geological, hydrological, biological, and atmospheric disciplines. Many of their resources and processes cannot be readily separated into simple categories. For example, evidence of microbiological roles in some speleogenesis (cave formation) and mineral deposition continues to mount (Barton and Luiszer, 2005; Barton et al., 2001; Northup and Lavoie, 2001). Are these microbiological processes best considered biological, geological, both, neither, or something in between? Cave climate, although probably best considered as an air resources issue, has very profound effects on many aspects of caves and cave resources. In fact, it may be the single most important aspect of many caves to monitor for resource protection. In general, I have attempted to discuss the main vital signs that can provide important information for managing caves as a whole or important geological resources within them. In the case of obviously hydrological vital signs, I have generally discussed them with less detail. Numerous agencies, including the National Park Service (NPS) Water Resources Division, U.S. Geological Survey Water Resources Program, the Environmental Protection Agency Office of Water, and various state agencies, can provide additional guidance on water monitoring, as well as access to hydrologists to assist in developing monitoring programs.
A related issue with cave monitoring is that caves contain a range of “geological settings,” some of which overlap with traditional surface geological settings that are discussed elsewhere in this book. Managers may find other chapters assist in developing monitoring programs for caves and cave resources. For example, a cave may have active streams or lakes that could be analyzed and monitored in ways at least similar to surface streams or lakes. Lava tubes are obviously volcanic features; some volcanic monitoring techniques may be applicable to at least the youngest lava tubes, such as those found on the island of Hawai’i. Caves may contain very significant paleontological resources; monitoring of these resources will assist in protecting them, but may also provide more general information about impacts to the individual caves.
VITAL SIGN: CAVE METEOROLOGY
Cave meteorology refers to the microclimate and air composition of the cave. It includes parameters such as air temperature, water vapor content of air (variously measured and expressed as absolute humidity, relative humidity, saturation debt, or dew point), evaporation rates, condensation, air movement (speed, direction, flux, etc.), barometric pressure, carbon dioxide level, radon level, and hazardous gas levels (methane, hydrogen sulfide, carbon monoxide). In addition to these air parameters, cave sediment temperature, water temperature, and rock temperature are commonly monitored with cave meteorology.
Cave meteorological parameters are among the most commonly monitored parameters in cave management. There are three basic reasons for this fact. First, many cave processes are very sensitive to changes in cave meteorological parameters. Microclimate can influence the form and morphology of the mineralogy and the crystal structure of speleothems (secondary mineralogical deposits that form in caves, e.g., stalactites and stalagmites) (Hill and Forti, 1997). Decreases in humidity can lead to a decline in cave-adapted animal life. For example, an increase in cave temperature may turn an ideal bat hibernaculum into a cave that is not used by bats. Increases in the concentration of carbon dioxide in cave air can lead to dissolution of cave formations. Paleontological, archaeological and cultural resources in a cave can be damaged by changes in cave microclimate. Second, cave meteorology is relatively sensitive to human activities in caves. The presence of people in a cave can significantly alter the microclimate of some low-energy flow caves, and large-scale tourist activities will alter the microclimate even in high-energy flow caves. Third, some of the cave meteorological parameters, such as temperature and evaporation, are among the most readily monitored cave parameters (at least at a very basic level).
It is difficult to generalize about the variability of cave meteorological parameters, both because of the difference in caves and because different parameters may show different patterns. In general, the variability of temperature, humidity, evaporation, condensation, and carbon dioxide levels is greater near the entrances to a cave. Deep in the cave these parameters may vary by only very small amounts (tenths of a degree in the case of air temperature, or fractions of a percent in the case of relative humidity). Sediment, water, and rock temperatures tend to be much less variable than air temperatures in the same area. Air flow (wind speed and direction) is much more difficult to generalize. Near entrances, significant airflow may be expected; however, in constricted areas deep in the cave, air flow may also be highly variable. In many cases, cave meteorological readings are best interpreted with surface meteorological readings from the same area.
Monitoring of cave microclimate may be needed for several different reasons. Because cave climate conditions are both broadly sensitive to and dependent on external climate conditions, monitoring cave climate conditions (especially in caves that are rarely visited) can provide information on natural regional climate change. More importantly, from a management perspective, monitoring helps to assess risk to resources due to cave climate changes (natural or artificial). In some caves, the presence of, amounts of, and variations in exotic gases (such as H2S, CO, CH4, etc.) are important to understanding the cave processes and to protecting people who may enter such caves. Because of the sensitivity of many cave resources to changes in cave microclimate, monitoring should take place when there are significant resources that could be impacted by changes in cave climate, when management actions might result in a change in climate in a cave, or to provide control data for a nearby cave undergoing a management
action.
When developing a cave meteorology monitoring program, it is important to consider both the range of values that can be anticipated for various parameters, and how much change in a variable will be significant in affecting cave processes, or likely to result from human impacts or management actions. This type of information would be obtained through an inventory process where a variety of parameters may be measured using either spot measurements or relatively short runs with data logging instruments. This type of inventory will help establish how the parameters vary in the cave over time. It will also provide important data that allows selection of the most appropriate instruments for measuring various parameters.
Radon concentration in cave air is an important parameter that is monitored in a number of caves (including many NPS show caves). Radon variation data can be used to examine cave airflow patterns (i.e., Pflitsch and Piasecki, 2003). However, most, if not all, radon monitoring is driven by regulatory requirements aimed at safeguarding human health. The NPS Air Resources Division is currently revising radon monitoring procedures for NPS caves (B. Carson, Mammoth Cave National Park, personal commun., 2008). In addition, the National Caves Association (the primary show caves industry group in the United States) has developed industry standards for monitoring and mitigating radon exposures in show caves (Aley et al., 2006).
Methods
Methods of monitoring cave meteorology vary from the extremely cheap and easy to the very expensive and complex. The main variable in cost is frequently the instruments used to measure and record the data. The more expensive instruments often provide greater accuracy and precision, greater storage
capacity and greater flexibility in timing of measurements. Cigna (2002) provides good information on a range of monitoring solutions for cave meteorology. The National Park Service’s Cumberland Piedmont Inventory and Monitoring Network has developed protocols for monitoring some aspects of cave meteorology, in particular air temperature, humidity, and airflow (J. Jernigan, Mammoth Cave National Park, personal commun., 2008). Copies of these protocols are available from the Cumberland Piedmont I&M Network (http://science.nature.nps.gov/im/units/cupn/). In addition, a group of resource management specialists from the NPS are working to expand those protocols to include additional aspects of cave meteorology. This work is scheduled to be completed in 2010.
When these protocols are finished, they will provide very useful guidance for monitoring these parameters. The protocols being developed focus mainly on mixed use of handheld instrumentation and datalogged sensors, and thus would generally fall in the medium level of cost, technology, and expertise. They also focus on developing an understanding of the spatial and temporal variation in parameters as part of developing a monitoring program.
In general, much basic monitoring can be accomplished by relatively inexpensive people, such as Student Conservation Association interns (SCAs), volunteers, citizen scientists, or students with some training using handheld instruments (such as thermometers, sling psychrometers, anemometers, or gas meters; Fig. 1). The timing between measurements will vary depending on the type of issues being examined. For deep cave measurements in caves not subjected to management actions that might alter cave climate, seasonal measurements may provide adequate data. If caves are subject to management actions that might alter climate (or if they serve as controls) more frequent measurement (monthly or weekly) might be appropriate. Because of variation in instruments, calibration is frequently necessary. Many relatively inexpensive thermometers can be calibrated against either a master thermometer or known temperature points (such as an ice and water mix). Cigna (2002) recommends 0.1 °C accuracy and precision for general purpose cave monitoring. Some specialized monitoring may require more accuracy and precision.
Evaporation and condensation are important meteorological processes that may affect cave resources. Evaporation rates may affect mineral growth rates and styles, desiccation potential of cave fauna, and cave pool extents and depths. Evaporation can be measured relatively easily with simple evaporation pans with standardized amounts of water. However, in areas near entrances, it may be important to protect evaporation pans from wildlife.
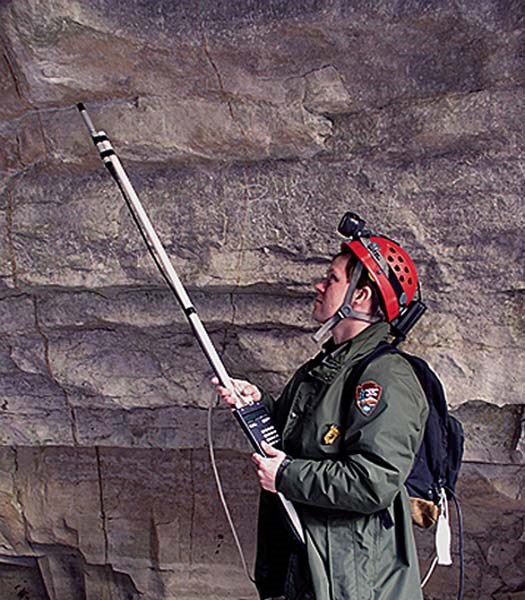
can measure condensation. De Freitas and Schmeckal (2005) developed a data logging sensor that is capable of detecting and (if properly calibrated) measuring condensation. Their sensor is fundamentally similar to leaf-wetness sensors that are now commercially available. These sensors measure changes in electrical resistance that occur due to water condensing on a circuit board.
The recommended technique for monitoring cave carbon dioxide levels is non-dispersive infrared spectroscopy (NDIR) (James, 2004). Handheld NDIR CO2 monitors are available for less than $1,000 (all amounts listed herein are in U.S. dollars). Spot and periodic monitoring of toxic gases in caves (such as H2S, CH4, CO, etc.) can be accomplished with stand-alone single or multi-gas detectors. Detectors can be purchased for between $500 and $2,000, depending on capabilities.
Automated data collection with either combined sensors and data loggers (such as HOBO brand data loggers) or specialized sensors attached to data loggers represent a medium level of complexity and expense. The data loggers tend to be somewhat more expensive than handheld instruments, although some temperature data loggers that can be readily used in caves are available for about $100 or less. Acquiring cave meteorological data using data loggers has several advantages over human recording using handheld instruments. In general, it is practical to take data more frequently with a data logger. In addition, measurement errors may be reduced. Data loggers are generally easy to use, maintain, and download. However, the large quantity of data that may result from a large network of data loggers may require staff with specialized data management experience.
The use of data loggers in caves is not as straightforward as it is in many surface applications. The high humidity and frequently condensing environment in caves leads to failure in many electronic devices over time. Finding data loggers that will work in caves for long-term application can be difficult. Only instruments rated for outdoor settings should be considered in any but the driest cave environments. In case of separate probes feeding data to a stand-alone data logger, it may be possible to place the logger in a case with drying agents that protect the logger from cave conditions. If you are considering using data loggers in a cave, contact other cave managers to find out what types of loggers have been used successfully in caves like the ones you are monitoring. In the case of caves with toxic, flammable, or corrosive gases, the longterm detection and logging can be even more difficult, because the instrumentation must survive not only cave conditions, but the additional challenges posed by the exotic gases.
Two cave meteorological parameters can be very difficult to measure precisely and accurately in cave conditions. These parameters are airborne moisture (humidity) and airflow (wind speed and directions). Because of this, these two parameters require more specialized approaches.
Humidity is difficult to measure at very high levels (above 95%). Unfortunately, many caves have relative humidities higher than this level. Thus, specialized, often expensive, instruments are needed to accurately measure cave humidity. Few if any of these specialized instruments are designed to function under the rigorous conditions of a cave. In addition, many of these instruments require signifi cant experience to obtain and interpret the results. One example of a potential technology for measuring humidity very accurately is laser absorption. Laser absorption measures the amount of loss of specific wavelengths as they pass through air. This absorption provides a very accurate measure of the amount of water vapor present in the air (absolute humidity). Unfortunately, this type of instrument is relatively expensive ($5,000–$10,000), and has not been tested in cave environments.
Less expensive instruments, like sling psychrometers and inexpensive electronic sensors, will provide general information on humidity changes, but are unlikely to provide truly accurate and precise monitoring.
Unlike humidity, airflow is not inherently difficult to measure in cave conditions. Practical problems do present more difficulty. Although some caves have very strong winds, many have much more gentle airflow or experience gentle airflow occasionally. In addition, depending on passage geometry and meteorological conditions, airflow may vary significantly in different parts of a passage cross section. In fact, airflow at the top of a passage may be in a different direction than that near the floor of the same passage. It is important to understand airflow variability in a cross section before choosing a place to monitor airflow. Further, the potential for a condensing environment causes measurement
problems. Anemometers based on physical movements (like cup or propeller anemometers) that are sensitive enough to measure small airfl ows are also subject to errors when condensation occurs on the mechanism. For this reason, hotwire and ultrasonic
anemometers are more appropriate for cave airflow studies and monitoring. Simple hotwire anemometers provide data on flow velocity, but not direction. However, they can be used in
pairs with one anemometer shielded to provide information on airflow direction as well (Cigna, 2004). Ultrasonic anemometers (Fig. 2) are beginning to be used to study and monitor cave airflow (Pflitsch and Piasecki, 2003). They have much promise for
improving monitoring, but they have some potential drawbacks. It is possible that ultrasonic anemometers could interfere with or disturb bats. In cases where bats may be an issue, anemometers that use frequencies higher than those used by area bats should
be strongly considered.
An inventory of expected values and level of change is especially important when dealing with difficult to measure parameters like relative humidity and airfl ow. For example, many relatively inexpensive instruments, data loggers, and probes will measure relative humidity; HOBO data loggers are a common type that are used in many monitoring situations. For many applications their thin film capacitance sensors provide usable levels of accuracy
(±2.5%) in environments with relative humidities between 10% and 90%. However, in environments with a relative humidity above 90%, their accuracy deteriorates to about ± 4% above 95% RH (Onset Computer Corporation, 2007). Thus, in caves or cave areas with fairly low relative humidities (below 90%), it is practical to use standard, inexpensive data loggers, especially if small changes in relative humidity are not likely to be of great significance. In high relative humidity situations or where changes in humidity of less than 2% are important, more expensive or elaborate methods may be more appropriate. Airflow is similar, in that the needed instruments and resolution may depend on what types and speeds of airflow occur in the cave.
Automated data collection with either combined sensors and data loggers (such as HOBO brand data loggers) or specialized sensors attached to data loggers represent a medium level of complexity and expense. The data loggers tend to be somewhat more expensive than handheld instruments, although some temperature data loggers that can be readily used in caves are available for about $100 or less. Acquiring cave meteorological data using data loggers has several advantages over human recording using handheld instruments. In general, it is practical to take data more frequently with a data logger. In addition, measurement errors may be reduced. Data loggers are generally easy to use, maintain, and download. However, the large quantity of data that may result from a large network of data loggers may require staff with specialized data management experience.
The use of data loggers in caves is not as straightforward as it is in many surface applications. The high humidity and frequently condensing environment in caves leads to failure in many electronic devices over time. Finding data loggers that will work in caves for long-term application can be difficult. Only instruments rated for outdoor settings should be considered in any but the driest cave environments. In case of separate probes feeding data to a stand-alone data logger, it may be possible to place the logger in a case with drying agents that protect the logger from cave conditions. If you are considering using data loggers in a cave, contact other cave managers to find out what types of loggers have been used successfully in caves like the ones you are monitoring. In the case of caves with toxic, flammable, or corrosive gases, the longterm detection and logging can be even more difficult, because the instrumentation must survive not only cave conditions, but the additional challenges posed by the exotic gases.
Two cave meteorological parameters can be very difficult to measure precisely and accurately in cave conditions. These parameters are airborne moisture (humidity) and airflow (wind speed and directions). Because of this, these two parameters require more specialized approaches.
Humidity is difficult to measure at very high levels (above 95%). Unfortunately, many caves have relative humidities higher than this level. Thus, specialized, often expensive, instruments are needed to accurately measure cave humidity. Few if any of these specialized instruments are designed to function under the rigorous conditions of a cave. In addition, many of these instruments require signifi cant experience to obtain and interpret the results. One example of a potential technology for measuring humidity very accurately is laser absorption. Laser absorption measures the amount of loss of specific wavelengths as they pass through air. This absorption provides a very accurate measure of the amount of water vapor present in the air (absolute humidity). Unfortunately, this type of instrument is relatively expensive ($5,000–$10,000), and has not been tested in cave environments.
Less expensive instruments, like sling psychrometers and inexpensive electronic sensors, will provide general information on humidity changes, but are unlikely to provide truly accurate and precise monitoring.
Unlike humidity, airflow is not inherently difficult to measure in cave conditions. Practical problems do present more difficulty. Although some caves have very strong winds, many have much more gentle airflow or experience gentle airflow occasionally. In addition, depending on passage geometry and meteorological conditions, airflow may vary significantly in different parts of a passage cross section. In fact, airflow at the top of a passage may be in a different direction than that near the floor of the same passage. It is important to understand airflow variability in a cross section before choosing a place to monitor airflow. Further, the potential for a condensing environment causes measurement
problems. Anemometers based on physical movements (like cup or propeller anemometers) that are sensitive enough to measure small airfl ows are also subject to errors when condensation occurs on the mechanism. For this reason, hotwire and ultrasonic
anemometers are more appropriate for cave airflow studies and monitoring. Simple hotwire anemometers provide data on flow velocity, but not direction. However, they can be used in
pairs with one anemometer shielded to provide information on airflow direction as well (Cigna, 2004). Ultrasonic anemometers (Fig. 2) are beginning to be used to study and monitor cave airflow (Pflitsch and Piasecki, 2003). They have much promise for
improving monitoring, but they have some potential drawbacks. It is possible that ultrasonic anemometers could interfere with or disturb bats. In cases where bats may be an issue, anemometers that use frequencies higher than those used by area bats should
be strongly considered.
An inventory of expected values and level of change is especially important when dealing with difficult to measure parameters like relative humidity and airfl ow. For example, many relatively inexpensive instruments, data loggers, and probes will measure relative humidity; HOBO data loggers are a common type that are used in many monitoring situations. For many applications their thin film capacitance sensors provide usable levels of accuracy
(±2.5%) in environments with relative humidities between 10% and 90%. However, in environments with a relative humidity above 90%, their accuracy deteriorates to about ± 4% above 95% RH (Onset Computer Corporation, 2007). Thus, in caves or cave areas with fairly low relative humidities (below 90%), it is practical to use standard, inexpensive data loggers, especially if small changes in relative humidity are not likely to be of great significance. In high relative humidity situations or where changes in humidity of less than 2% are important, more expensive or elaborate methods may be more appropriate. Airflow is similar, in that the needed instruments and resolution may depend on what types and speeds of airflow occur in the cave.
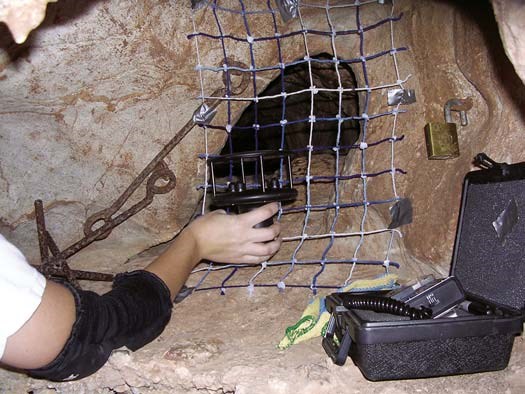
(Photo by R.S. Toomey.)
often less expensive, easier to use, and more durable, may be used for the monitoring.
In some cases, very small relative humidity perturbations (less than 1%) may have significant effects on cave processes and cave resources. Often these effects are more pronounced when equilibrium relative humidities are near saturation (100%). Numerous geologically and biologically significant processes in caves vary in form, magnitude, and direction within the relative humidity range of 90% to 100%. These include evaporation rates (Buecher, 1999), mineral stability (Hill and Forti, 1997; Onac and Vereş, 2003), and the loss or gain of biological water (Culver, 1982; Howarth, 1983). If cave relative humidities are in this upper range and these types of cave resources are present, the ability to distinguish humidities within this range may be necessary to adequately understand, anticipate, and possibly mitigate (if necessary) changes in these processes. However, if the caves being monitored are outside these high humidity ranges or do not contain resources sensitive to small humidity changes, then less expensive, easier to use instruments may be sufficiently accurate and precise to detect important changes.
Calibration can be a problem with many sensors over long periods of time in cave environments. To address the problem of calibration and to make sure measurements remain comparable over long periods, less expensive data logging sensors can be used for taking measurements, and more accurate handheld instruments can be used periodically to calibrate the data logging sensors. This can also be cost effective, because fewer expensive, high accuracy instruments are needed.
More expensive and complex cave meteorology monitoring programs will use increasingly complex and specialized sensors attached to networks of data loggers. These sensors might include very sensitive thermometers, complex psychrometers, ultrasonic anemometers, and gas chromatographs (for analyzing cave atmospheric composition). These systems will require very specialized knowledge to develop, maintain, and analyze the resulting data. Developing this level of cave meteorological monitoring will probably require partnerships with academic institutions or groups that have experience with this type of monitoring.
Calibration can be a problem with many sensors over long periods of time in cave environments. To address the problem of calibration and to make sure measurements remain comparable over long periods, less expensive data logging sensors can be used for taking measurements, and more accurate handheld instruments can be used periodically to calibrate the data logging sensors. This can also be cost effective, because fewer expensive, high accuracy instruments are needed.
More expensive and complex cave meteorology monitoring programs will use increasingly complex and specialized sensors attached to networks of data loggers. These sensors might include very sensitive thermometers, complex psychrometers, ultrasonic anemometers, and gas chromatographs (for analyzing cave atmospheric composition). These systems will require very specialized knowledge to develop, maintain, and analyze the resulting data. Developing this level of cave meteorological monitoring will probably require partnerships with academic institutions or groups that have experience with this type of monitoring.
Buecher (1999) discusses a variety of microclimate characterization and monitoring approaches that were used in predevelopment studies at Kartchner Caverns State Park, Arizona. These studies provide the basis for the ongoing monitoring there. One of the tools used for monitoring at Kartchner Caverns is an Environmental Monitoring Station (Fig. 3). These are permanently located stations at which temperature (air, water, and sediment), relative humidity, pan evaporation, and carbon dioxide are taken at regular intervals (weekly for most stations and monthly for more remote stations) by Arizona State Parks staff. In addition, many of these stations have HOBO temperature data loggers for taking measurements between readings. The weekly readings with calibrated thermometers allow for calibration of the HOBO data. These stations have been in use at Kartchner Caverns since 1989. Toomey and Nolan (2005) discuss the continuing monitoring of microclimate at Kartchner Caverns with respect to cave development, tours, and regional climate patterns; they provide a strong argument for including regional data in understanding the results of monitoring.
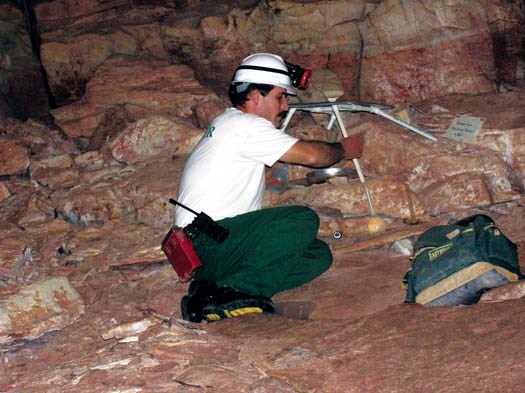
VITAL SIGN: AIRBORNE SEDIMENTATION
The sedimentation of dust and lint in caves is a significant issue for cave protection, especially in show caves. Natural sedimentation of dust can occur in caves due to natural cave processes such as airflow. However, when caves are visited, the natural level of dust can be supplemented by additional dust (mineral component) and lint (hair, skin cells, and clothing fibers) due to human activity. Monitoring dust and lint in caves that have sizeable visitation or sensitive resources is important because increased deposition can have profound effects on caves. Management actions can increase or reduce deposition. Dust deposition can alter and dull the color of cave formations; dust and lint can also alter the cave life. The deposition of organic materials (like lint) can increase food levels in the cave; the increases in food can allow the cave to be invaded by less cave-adapted forms and displace cave adapted organisms. In addition, decomposition of organic lint on formations can lead to corrosion of the formation surfaces. Jablonsky et al. (1995) and Michie (2001, 2004) provide good summaries of the issues involved with dust and lint in caves.
Methods
Dust and lint can be monitored by examining either the material deposited or the material in the air. In both cases, monitoring both the amount and composition of the dust is most desirable. In almost all cases, comprehensive monitoring of dust will require both specialized training and specialized equipment.
Level 1/2 Methods
General characterization of lint removal activities. If lint clean-up occurs at a cave, it would be desirable to quantify and characterize the lint that is collected in the clean-up. This can vary from simply weighing the lint that is collected to using microscopes and higher tech chemical means to characterize the lint content. The level of training and equipment needed depends on what detail of lint identification is desired. When using these approaches, it is important to standardize for different amounts of collecting effort in different areas or years.
The sedimentation of dust and lint in caves is a significant issue for cave protection, especially in show caves. Natural sedimentation of dust can occur in caves due to natural cave processes such as airflow. However, when caves are visited, the natural level of dust can be supplemented by additional dust (mineral component) and lint (hair, skin cells, and clothing fibers) due to human activity. Monitoring dust and lint in caves that have sizeable visitation or sensitive resources is important because increased deposition can have profound effects on caves. Management actions can increase or reduce deposition. Dust deposition can alter and dull the color of cave formations; dust and lint can also alter the cave life. The deposition of organic materials (like lint) can increase food levels in the cave; the increases in food can allow the cave to be invaded by less cave-adapted forms and displace cave adapted organisms. In addition, decomposition of organic lint on formations can lead to corrosion of the formation surfaces. Jablonsky et al. (1995) and Michie (2001, 2004) provide good summaries of the issues involved with dust and lint in caves.
Methods
Dust and lint can be monitored by examining either the material deposited or the material in the air. In both cases, monitoring both the amount and composition of the dust is most desirable. In almost all cases, comprehensive monitoring of dust will require both specialized training and specialized equipment.
Level 1/2 Methods
General characterization of lint removal activities. If lint clean-up occurs at a cave, it would be desirable to quantify and characterize the lint that is collected in the clean-up. This can vary from simply weighing the lint that is collected to using microscopes and higher tech chemical means to characterize the lint content. The level of training and equipment needed depends on what detail of lint identification is desired. When using these approaches, it is important to standardize for different amounts of collecting effort in different areas or years.
Level 2/3 Methods
Analysis of dust and/or lint collecting plates. Collecting dust and lint to measure deposition rates is generally straightforward. A series of glass plates (or Petri dishes) are placed in the cave to collect falling dust. They can be placed in transects to look at trends in dust deposition or as single plates to look at spot deposition. Plates are left in the cave for set amounts of time. The amount of time that plates should be left varies with deposition rate, the goal of the study (general monitoring or testing a particular management strategy), and sensitivity of the method of quantifying dust deposition. Frequently, multiple plates or dishes are placed together, so that individual plates may be analyzed at different intervals (Fig. 4). The plates are then removed from the cave and the amount and types of dust and lint are determined. Plate collection provides integrated information on dust and lint deposition over a known time period.
Analysis of dust and/or lint collecting plates. Collecting dust and lint to measure deposition rates is generally straightforward. A series of glass plates (or Petri dishes) are placed in the cave to collect falling dust. They can be placed in transects to look at trends in dust deposition or as single plates to look at spot deposition. Plates are left in the cave for set amounts of time. The amount of time that plates should be left varies with deposition rate, the goal of the study (general monitoring or testing a particular management strategy), and sensitivity of the method of quantifying dust deposition. Frequently, multiple plates or dishes are placed together, so that individual plates may be analyzed at different intervals (Fig. 4). The plates are then removed from the cave and the amount and types of dust and lint are determined. Plate collection provides integrated information on dust and lint deposition over a known time period.
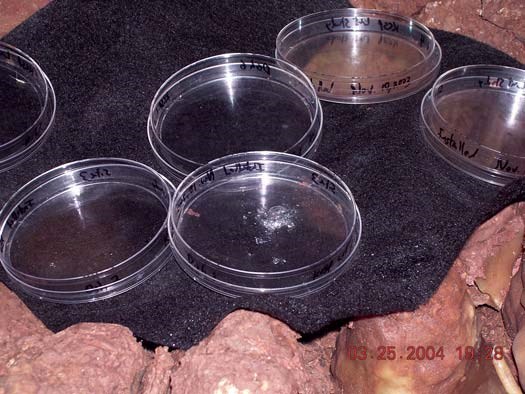
(Photo by R.S. Toomey.)
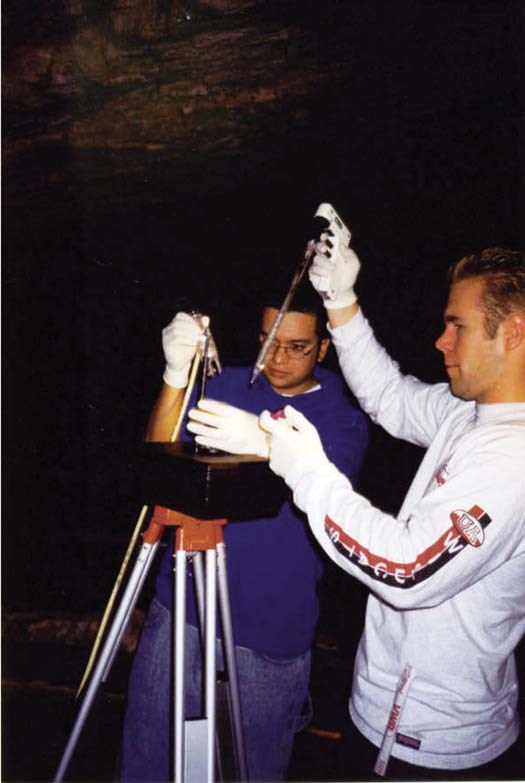
or chromatography). The field work to deploy or collect plates can be accomplished by volunteers and only inexpensive equipment is used in the field. However, the analysis of the collected plates requires both specialized equipment and trained scientists. The method is generally a Level 2 or 3 method, depending on the level of identification of sedimentation components and methods used to identify components.
Level 2/3 Methods
Analysis of airborne dust/lint. Measuring airborne dust and lint in caves is general accomplished by one of two methods. In one method, air can be drawn through a filter and the material trapped on the filter can be analyzed. Dry vacuum filters and impingers (Fig. 5) are two different types of these filters. Methods of analysis include microscopic counting and identification and collecting for weighing and chemical analysis. Another method uses optical means (such as lasers) to measure the dispersion of light caused by airborne dust. Some types of laser measurement devices (such as aerodynamic particle sizers) can determine both the number and size distribution of airborne particles (Michie, 2004). As with the plate collection methods, airborne particle characterization requires both medium level technology and specialists to accomplish. Unlike plate collection techniques, airborne sampling provides information on the amount of dust in the air at a specific time, as opposed to time-averaged rates of sedimentation.
VITAL SIGN: DIRECT VISITOR IMPACTS
Direct impacts of cavers and tourists are the most important manageable source of loss and damage of cave resources. Direct impacts include such impacts as breakage of cave formations, development and expansion of trails in caves, damage to cave surfaces from traffic, and graffiti or similar damage. Impacts such as these result in direct loss and damage to cave resources (including geological, biological and cultural resources). Monitoring can help managers to understand patterns of impact, devise strategies to limit impact, and determine whether and how management actions change impacts. Related to monitoring of visitor impacts is monitoring visitor traffic in caves. Another direct impact that can occur in caves with fixed lighting is the growth of “lamp flora.”
Unlike some other types of cave monitoring, most regularly used visitor impact monitoring methods require only minimal technology. However, some require specially trained staff to accurately assess impact.
Methods—Visitor Numbers
Level 1 Methods
Visitor counts (tickets, permits, cave registers). Monitoring the number of visitors to caves seems like a simple task, and indeed it can be. In show caves, recording the number of people on the tours can provide this basic information. In wild caves, monitoring the number of visitors can be more difficult. Cave registers are in-cave forms on which cave visitors record such information as their names, ages, home cities, affiliations, and party size. Filling these out is voluntary, so the forms do not necessarily provide complete, accurate information. Placing multiple registers in different areas of a cave can provide some data on relative visitation in different areas. Caving permit systems, where cavers are required to get permits to visit caves, provide an additional estimate of cave visitation. Estimates will be more accurate for caves where access is restricted by a gate or other barrier, because gates should limit the number of non-permitted entries. These techniques are all very easy ones to implement with volunteers or inexpensive paid personnel; they also require only inexpensive materials.
Visitor counts (data loggers). Another approach to monitoring visitation in non-tour caves is to use some type of data logger (or automatic counter) to count groups and individuals visiting caves. Several groups have developed techniques that can be used to monitor visitation. Most of these techniques have focused on using the presence of light in normally dark areas of the cave to detect and log visits (Drummond, 1992; Gibson, 1994; Johnson
et al., 2002; and Toomey et al., 2001). Some have used data loggers that record when an infrared beam is broken. Other types of trail counters (including pressure pads and air-tube counters) have the potential to be used for monitoring cave visitation, but reports do not suggest that these have been used frequently in caves. Unfortunately, calibration of all of these types of counters has proven diffi cult. They provide a count of events, rather than of individual visitors. At this point they are better suited to provide information on timing of cave use and general patterns of visitation than accurate visitation numbers. Overall, this approach to monitoring visitation is relatively easy to accomplish with inexpensive personnel and materials (less than $500).
Methods—Direct Impact Assessment
Several techniques can be used to monitor direct visitor impacts, such as cave formation breakage, trail development, and trail compaction. Five techniques discussed here are photomonitoring, formation breakage counts, visitor impact mapping, trail compaction monitoring, and light detection and ranging (LIDAR) scanning.
These methods vary due to the wide range of impacts measured. Many of the methods do not require specialized equipment and can be accomplished with specialized volunteer labor. However, most are quite labor intensive, especially if the area of cave that must be assessed is large or complex. For this reason, most methods are classified as level two.
Level 1 Methods
“Lamp flora” mapping. In show caves with fixed lighting, algae, cyanobacteria (commonly called blue-green algae), and higher plants may grow on moist cave surfaces that are brightly lit. This invasive plant growth, known as “lamp flora” can significantly impact cave geological, biological, and cultural resources. A discussion of techniques to limit or control the growth of lamp flora is well beyond the scope of this discussion. Aley (2004) and Olson (2006) provide a good introduction to the subject. In caves where lamp flora occurs, it is important to monitor both the growth of lamp flora and the actions taken to control it. Monitoring growth of lamp flora is relatively simple. Such monitoring may consist of photomonitoring areas of growth, or manually mapping or recording sites of growth. Manually mapping of algal growth areas can be accomplished for relatively little cost with volunteer or inexpensive paid labor. It does not require any specialized equipment.
Monitoring changes in what organisms make up lamp flora growth is much more involved and expensive. Doing so will require working with experts in algae. In general, this kind of specialized study is beyond the scope of “lamp flora” monitoring studies.
Sediment compaction. When sediment floors of a cave are traversed, the development of trails and associated trail compaction almost invariably occurs. Such compaction can be a significant impact on the cave sediments. It can also impact other cave resources, such as archaeological or paleontological materials contained in the sediment fill. Compacted sediments may also inhibit the movement of some cave animals and may alter or prevent the growth of some types of cave minerals that grow out of the sediments. Sediment compaction can be easily monitored with trained volunteers, students, or employees using fairly inexpensive equipment. A soil compaction tester or penetrometer can be used to map the soil compaction in trails and in adjacent non-trail areas. Monitoring trail compaction can be used to adjust limits on numbers of visitors and may be used to limit access to impacted areas. In some types of sediments, bioturbation (churning of sediments by animals) and mineral growth can reverse compaction if travel is halted or reduced. Although monitoring of sediment compaction is listed here as Level 1 monitoring, the complex nature of cave sediment floors can make interpretation of compaction patterns and changes quite complex. Initial study design may require assistance of a soil scientist (J. Roth, 2006, personal commun.).
Level 2 Methods
Photomonitoring. Photomonitoring is a very important technique for a range of condition monitoring in caves and other environments. It is so important that it is covered in a separate chapter. However, photomonitoring in caves can be a somewhat challenging. Werker and Hildreth-Werker (1996) and Hildreth-Werker (2006) provide many useful suggestions for improving photomonitoring of the cave environment. The amount of specialized equipment needed for cave photomonitoring is limited. Specialized permanent mounting systems have been developed to allow easy repetition of monitoring photos (Werker and Hildreth-Werker, 1996; Hildreth-Werker, 2006) for areas where monitoring will be relatively frequent; these mounts are generally inexpensive. Photomonitoring in caves can be, and often is, accomplished by volunteers. However, these volunteers must be either well trained or experienced in the techniques.
Analysis of airborne dust/lint. Measuring airborne dust and lint in caves is general accomplished by one of two methods. In one method, air can be drawn through a filter and the material trapped on the filter can be analyzed. Dry vacuum filters and impingers (Fig. 5) are two different types of these filters. Methods of analysis include microscopic counting and identification and collecting for weighing and chemical analysis. Another method uses optical means (such as lasers) to measure the dispersion of light caused by airborne dust. Some types of laser measurement devices (such as aerodynamic particle sizers) can determine both the number and size distribution of airborne particles (Michie, 2004). As with the plate collection methods, airborne particle characterization requires both medium level technology and specialists to accomplish. Unlike plate collection techniques, airborne sampling provides information on the amount of dust in the air at a specific time, as opposed to time-averaged rates of sedimentation.
VITAL SIGN: DIRECT VISITOR IMPACTS
Direct impacts of cavers and tourists are the most important manageable source of loss and damage of cave resources. Direct impacts include such impacts as breakage of cave formations, development and expansion of trails in caves, damage to cave surfaces from traffic, and graffiti or similar damage. Impacts such as these result in direct loss and damage to cave resources (including geological, biological and cultural resources). Monitoring can help managers to understand patterns of impact, devise strategies to limit impact, and determine whether and how management actions change impacts. Related to monitoring of visitor impacts is monitoring visitor traffic in caves. Another direct impact that can occur in caves with fixed lighting is the growth of “lamp flora.”
Unlike some other types of cave monitoring, most regularly used visitor impact monitoring methods require only minimal technology. However, some require specially trained staff to accurately assess impact.
Methods—Visitor Numbers
Level 1 Methods
Visitor counts (tickets, permits, cave registers). Monitoring the number of visitors to caves seems like a simple task, and indeed it can be. In show caves, recording the number of people on the tours can provide this basic information. In wild caves, monitoring the number of visitors can be more difficult. Cave registers are in-cave forms on which cave visitors record such information as their names, ages, home cities, affiliations, and party size. Filling these out is voluntary, so the forms do not necessarily provide complete, accurate information. Placing multiple registers in different areas of a cave can provide some data on relative visitation in different areas. Caving permit systems, where cavers are required to get permits to visit caves, provide an additional estimate of cave visitation. Estimates will be more accurate for caves where access is restricted by a gate or other barrier, because gates should limit the number of non-permitted entries. These techniques are all very easy ones to implement with volunteers or inexpensive paid personnel; they also require only inexpensive materials.
Visitor counts (data loggers). Another approach to monitoring visitation in non-tour caves is to use some type of data logger (or automatic counter) to count groups and individuals visiting caves. Several groups have developed techniques that can be used to monitor visitation. Most of these techniques have focused on using the presence of light in normally dark areas of the cave to detect and log visits (Drummond, 1992; Gibson, 1994; Johnson
et al., 2002; and Toomey et al., 2001). Some have used data loggers that record when an infrared beam is broken. Other types of trail counters (including pressure pads and air-tube counters) have the potential to be used for monitoring cave visitation, but reports do not suggest that these have been used frequently in caves. Unfortunately, calibration of all of these types of counters has proven diffi cult. They provide a count of events, rather than of individual visitors. At this point they are better suited to provide information on timing of cave use and general patterns of visitation than accurate visitation numbers. Overall, this approach to monitoring visitation is relatively easy to accomplish with inexpensive personnel and materials (less than $500).
Methods—Direct Impact Assessment
Several techniques can be used to monitor direct visitor impacts, such as cave formation breakage, trail development, and trail compaction. Five techniques discussed here are photomonitoring, formation breakage counts, visitor impact mapping, trail compaction monitoring, and light detection and ranging (LIDAR) scanning.
These methods vary due to the wide range of impacts measured. Many of the methods do not require specialized equipment and can be accomplished with specialized volunteer labor. However, most are quite labor intensive, especially if the area of cave that must be assessed is large or complex. For this reason, most methods are classified as level two.
Level 1 Methods
“Lamp flora” mapping. In show caves with fixed lighting, algae, cyanobacteria (commonly called blue-green algae), and higher plants may grow on moist cave surfaces that are brightly lit. This invasive plant growth, known as “lamp flora” can significantly impact cave geological, biological, and cultural resources. A discussion of techniques to limit or control the growth of lamp flora is well beyond the scope of this discussion. Aley (2004) and Olson (2006) provide a good introduction to the subject. In caves where lamp flora occurs, it is important to monitor both the growth of lamp flora and the actions taken to control it. Monitoring growth of lamp flora is relatively simple. Such monitoring may consist of photomonitoring areas of growth, or manually mapping or recording sites of growth. Manually mapping of algal growth areas can be accomplished for relatively little cost with volunteer or inexpensive paid labor. It does not require any specialized equipment.
Monitoring changes in what organisms make up lamp flora growth is much more involved and expensive. Doing so will require working with experts in algae. In general, this kind of specialized study is beyond the scope of “lamp flora” monitoring studies.
Sediment compaction. When sediment floors of a cave are traversed, the development of trails and associated trail compaction almost invariably occurs. Such compaction can be a significant impact on the cave sediments. It can also impact other cave resources, such as archaeological or paleontological materials contained in the sediment fill. Compacted sediments may also inhibit the movement of some cave animals and may alter or prevent the growth of some types of cave minerals that grow out of the sediments. Sediment compaction can be easily monitored with trained volunteers, students, or employees using fairly inexpensive equipment. A soil compaction tester or penetrometer can be used to map the soil compaction in trails and in adjacent non-trail areas. Monitoring trail compaction can be used to adjust limits on numbers of visitors and may be used to limit access to impacted areas. In some types of sediments, bioturbation (churning of sediments by animals) and mineral growth can reverse compaction if travel is halted or reduced. Although monitoring of sediment compaction is listed here as Level 1 monitoring, the complex nature of cave sediment floors can make interpretation of compaction patterns and changes quite complex. Initial study design may require assistance of a soil scientist (J. Roth, 2006, personal commun.).
Level 2 Methods
Photomonitoring. Photomonitoring is a very important technique for a range of condition monitoring in caves and other environments. It is so important that it is covered in a separate chapter. However, photomonitoring in caves can be a somewhat challenging. Werker and Hildreth-Werker (1996) and Hildreth-Werker (2006) provide many useful suggestions for improving photomonitoring of the cave environment. The amount of specialized equipment needed for cave photomonitoring is limited. Specialized permanent mounting systems have been developed to allow easy repetition of monitoring photos (Werker and Hildreth-Werker, 1996; Hildreth-Werker, 2006) for areas where monitoring will be relatively frequent; these mounts are generally inexpensive. Photomonitoring in caves can be, and often is, accomplished by volunteers. However, these volunteers must be either well trained or experienced in the techniques.
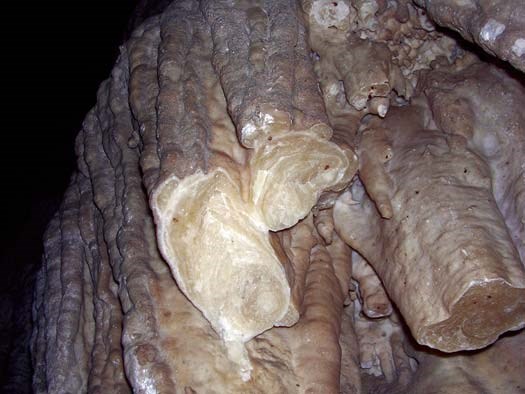
Formation breakage counts. Formation breakage counts are a very simple means to monitor breakage of cave formations. In this technique, an inventory is made of broken formations in a cave (or area of a cave). All broken formations identified in this inventory are inconspicuously marked. In a study in Carlsbad Caverns (D. Pate, 2005, personal commun.), small red ink dots were placed on each broken formation as it was inventoried
(Fig. 6). Broken formations are re-inventoried at intervals and broken formations without red dots are counted as new breakage. An alternative that would allow for larger or more conspicuously placed dots would be to use indelible ink that reflects only in the ultraviolet range. Oregon Caves National Monument is experimenting with the use of such inks for marking previously broken formations (Hale, 2007). Repeated formation breakage counts are a very low-tech way to monitor the breakage of formations in both show and wild caves. In addition, the technique does not require highly trained staff. However, in a highly decorated cave, anything more than a very small area can take many hours to monitor. In addition, this technique works better with larger speleothems like stalactites and draperies, than with smaller or more complex speleothems such as coralloids or helectites. Overall, although this technique is inexpensive and can be accomplished with volunteers, it is usually quite labor intensive. For this reason, it has been classifi ed as a Level 2 technique.
Visitor impact mapping and inventory. Visitor impact mapping is another technique to monitor human impacts on caves and cave resources. Bodenhamer (1996, 2006) developed this technique as a way to quantify and monitor impacts such as formation
breakage and floor surface impacts. In visitor impact mapping, a cave or cave area is examined for visitor impacts (such as compaction and trails, sediment disturbance, mud transferred to clean surfaces, broken floor crusts, and broken formations) and these impacts are mapped onto a detailed map of the cave. The technique, a specialized form of cave mapping, does not require specialized technology. It does, however, require cavers skilled in cave mapping and trained in the technique. It also requires detailed base maps of the cave or cave area; Bodenhamer (2006) suggests that cave mapping with a minimum scale of 1:240 (1 inch equals 20 ft or ~1 cm equals 2.5 m) provides a good base map. Initial visitor impact mapping takes about the same amount of time as setting up a photomonitoring transect; however, repeat mapping to assess impacts is usually completed more quickly (Bodenhamer 2006). Allison (2004) describes the application of this technique
in Lechuguilla Cave, Carlsbad Caverns National Park.
Oregon Cave National Monument has taken a related, but different approach to visitor impact mapping (Hale, 2007). Rather than mapping the impacts using the Bodenhamer (2006) method, they inventory impacts using 29 categories of impacts that can be readily identified and classified. The inventory is made using a portable digital assistant using ArcPad. The integration of the inventory into cave geographic information system (GIS) data
sets assists in the analysis of impacts.
Impact mapping does not require specialized equipment. However, it does require highly trained volunteers or specialists. In most cases, these specialists or volunteers are skilled cavers who map visitor impacts to assist management agencies. The fact that the process is often quite labor intensive, especially in large or complex cave areas means that this is usually a Level 2 monitoring method.
Level 3 Methods
LIDAR or time-of-flight laser scanning. In very sensitive areas with unique, rare or valuable resources, LIDAR (light detection and ranging) or time-of-flight laser scanning can be used to monitor changes in the area. This is a technique that requires very expensive technology and highly specialized personnel. In this technique, a laser scanner is used to map a cave area in great detail. A laser is scanned across the area and the distance to each point in the room is measured to a high degree of precision and accuracy. These distances are used to develop a “point cloud” that maps the room in three dimensions. Repeated scanning at intervals can be used to monitor even small changesin cave resources. This technique, although undoubtedly powerful, currently has a number of limitations in addition to cost and personnel needs when it is applied to caves. Depending on the setup, precisions near one millimeter are possible; however, even at these resolutions, the types of visitor impacts that can be detected are limited to relatively large impacts such as broken cave formations. The scanning units are relatively large, heavy, and delicate (Fig. 7). In addition, they require either large batteries or electrical connections. While they may be useful in show caves, these methods probably have limited application to backcountry wild caves.
VITAL SIGN: PERMANENT OR SEASONAL ICE
Some caves at high latitudes or high elevations have either permanent or seasonal ice. This ice is important for several reasons. First, it helps control the cave microclimate. Second, it can contain a wealth of paleoclimatic data (material such as pollen trapped in the ice, an isotope record of climate, etc). The extent of ice in caves can itself be used to interpret climate changes (Luetscher, et al., 2005). Yonge (2004) discusses the characteristics of ice in caves and different mechanisms which lead to seasonal and permanent ice.
Natural variation in climate (in particular, local and regional variations in precipitation and temperature) can affect the extent of ice in caves with such ice. In addition, management actions, such as land use changes or entrance modifications, may also affect ice extent (thickness, area, or temporality). Monitoring ice extent may provide information on both long-terms changes in climate and the effects of management actions.
Ice volume in caves may be very sensitive to seasonal conditions. For this reason it is very important for monitoring to take place during the same season or seasons each year. An alternative approach is to measure thickness or extent when it is at its minimum each year; such measurements may represent a better indication of net annual effects (J. Roth, 2006, personal commun.).
Methods
Level 1 Methods
Manual measuring of ice thickness or extent. Ice extent and thickness can generally be monitored using inexpensive means that do not require specialized personnel. The extent of ice can be readily mapped using several techniques. Mapping with respect to set landmarks can be accomplished using a detailed map of the cave area, simple survey instruments, a prepared set of landmarks, or an established grid.
(Fig. 6). Broken formations are re-inventoried at intervals and broken formations without red dots are counted as new breakage. An alternative that would allow for larger or more conspicuously placed dots would be to use indelible ink that reflects only in the ultraviolet range. Oregon Caves National Monument is experimenting with the use of such inks for marking previously broken formations (Hale, 2007). Repeated formation breakage counts are a very low-tech way to monitor the breakage of formations in both show and wild caves. In addition, the technique does not require highly trained staff. However, in a highly decorated cave, anything more than a very small area can take many hours to monitor. In addition, this technique works better with larger speleothems like stalactites and draperies, than with smaller or more complex speleothems such as coralloids or helectites. Overall, although this technique is inexpensive and can be accomplished with volunteers, it is usually quite labor intensive. For this reason, it has been classifi ed as a Level 2 technique.
Visitor impact mapping and inventory. Visitor impact mapping is another technique to monitor human impacts on caves and cave resources. Bodenhamer (1996, 2006) developed this technique as a way to quantify and monitor impacts such as formation
breakage and floor surface impacts. In visitor impact mapping, a cave or cave area is examined for visitor impacts (such as compaction and trails, sediment disturbance, mud transferred to clean surfaces, broken floor crusts, and broken formations) and these impacts are mapped onto a detailed map of the cave. The technique, a specialized form of cave mapping, does not require specialized technology. It does, however, require cavers skilled in cave mapping and trained in the technique. It also requires detailed base maps of the cave or cave area; Bodenhamer (2006) suggests that cave mapping with a minimum scale of 1:240 (1 inch equals 20 ft or ~1 cm equals 2.5 m) provides a good base map. Initial visitor impact mapping takes about the same amount of time as setting up a photomonitoring transect; however, repeat mapping to assess impacts is usually completed more quickly (Bodenhamer 2006). Allison (2004) describes the application of this technique
in Lechuguilla Cave, Carlsbad Caverns National Park.
Oregon Cave National Monument has taken a related, but different approach to visitor impact mapping (Hale, 2007). Rather than mapping the impacts using the Bodenhamer (2006) method, they inventory impacts using 29 categories of impacts that can be readily identified and classified. The inventory is made using a portable digital assistant using ArcPad. The integration of the inventory into cave geographic information system (GIS) data
sets assists in the analysis of impacts.
Impact mapping does not require specialized equipment. However, it does require highly trained volunteers or specialists. In most cases, these specialists or volunteers are skilled cavers who map visitor impacts to assist management agencies. The fact that the process is often quite labor intensive, especially in large or complex cave areas means that this is usually a Level 2 monitoring method.
Level 3 Methods
LIDAR or time-of-flight laser scanning. In very sensitive areas with unique, rare or valuable resources, LIDAR (light detection and ranging) or time-of-flight laser scanning can be used to monitor changes in the area. This is a technique that requires very expensive technology and highly specialized personnel. In this technique, a laser scanner is used to map a cave area in great detail. A laser is scanned across the area and the distance to each point in the room is measured to a high degree of precision and accuracy. These distances are used to develop a “point cloud” that maps the room in three dimensions. Repeated scanning at intervals can be used to monitor even small changesin cave resources. This technique, although undoubtedly powerful, currently has a number of limitations in addition to cost and personnel needs when it is applied to caves. Depending on the setup, precisions near one millimeter are possible; however, even at these resolutions, the types of visitor impacts that can be detected are limited to relatively large impacts such as broken cave formations. The scanning units are relatively large, heavy, and delicate (Fig. 7). In addition, they require either large batteries or electrical connections. While they may be useful in show caves, these methods probably have limited application to backcountry wild caves.
VITAL SIGN: PERMANENT OR SEASONAL ICE
Some caves at high latitudes or high elevations have either permanent or seasonal ice. This ice is important for several reasons. First, it helps control the cave microclimate. Second, it can contain a wealth of paleoclimatic data (material such as pollen trapped in the ice, an isotope record of climate, etc). The extent of ice in caves can itself be used to interpret climate changes (Luetscher, et al., 2005). Yonge (2004) discusses the characteristics of ice in caves and different mechanisms which lead to seasonal and permanent ice.
Natural variation in climate (in particular, local and regional variations in precipitation and temperature) can affect the extent of ice in caves with such ice. In addition, management actions, such as land use changes or entrance modifications, may also affect ice extent (thickness, area, or temporality). Monitoring ice extent may provide information on both long-terms changes in climate and the effects of management actions.
Ice volume in caves may be very sensitive to seasonal conditions. For this reason it is very important for monitoring to take place during the same season or seasons each year. An alternative approach is to measure thickness or extent when it is at its minimum each year; such measurements may represent a better indication of net annual effects (J. Roth, 2006, personal commun.).
Methods
Level 1 Methods
Manual measuring of ice thickness or extent. Ice extent and thickness can generally be monitored using inexpensive means that do not require specialized personnel. The extent of ice can be readily mapped using several techniques. Mapping with respect to set landmarks can be accomplished using a detailed map of the cave area, simple survey instruments, a prepared set of landmarks, or an established grid.
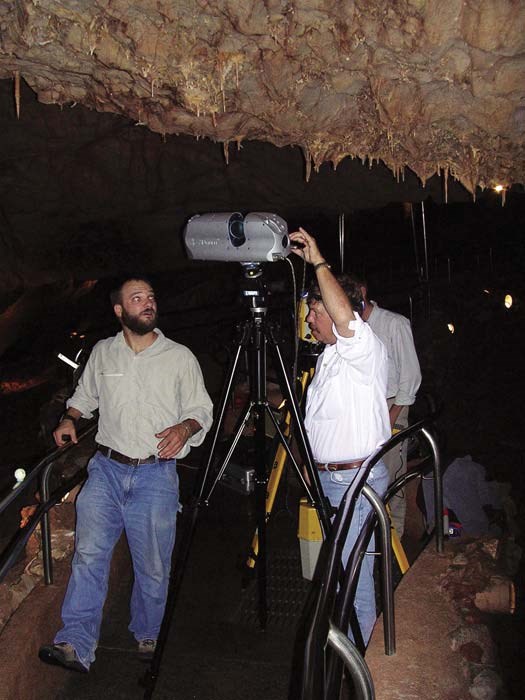
Ice extents and thicknesses have been monitored at Lava Beds National Monument since the 1970s (W. Devereaux, 2005, written commun.). In 2005, ice levels were monitored in nine caves. Monitoring of levels is performed by volunteers who measure the distance from the ice to permanent datums marked with stainless steel screws in each cave. The distance to both water on top of ice and to the ice itself are recorded. In addition, the condition of the ice, the presence of dead animals on the ice, and the condition of the rooms with ice are noted.
Level 2 Methods
Photomonitoring. Repeat photography is another method for monitoring ice extent, although with very clear ice, it may be difficult to discern ice extent in photographs. Historical photographs may be used to estimate past ice volumes and extend the monitoring record (Luetscher, et al., 2005). Werker and Hildreth-Werker (1996) and Hildreth-Werker (2006) provide many useful suggestions for improving photomonitoring of the cave environment.
Level 3 Methods
LIDAR or time-of-fl ight laser scanning. Repeat laser scanning can track ice extent accurately; however, the technique requires expensive equipment and highly trained specialists for data acquisition and reduction. A more complete discussion of
the limitations of this technique can be found in the Direct Visitor Impacts section above.
VITAL SIGN: CAVE DRIP AND POOL WATER
Dripping and pooled water are important components of many cave environments. Dripping water transports minerals, microbes, and chemicals into the cave. It is responsible for depositing minerals, resulting in the growth of many types of cave formations, most notably stalactites and stalagmites (Hill and Forti, 1997). Pooled water formed by infiltration (through dripping or flowing) is also responsible for the formation of several types of formations, such as shelfstone, rafts, pool fingers, and pool spar (Hill and Forti, 1997). Dripping and pooled water also acts to maintain cave moisture. Drip water can also transport contaminants into caves.
Because of its crucial roles in depositing cave formations and maintaining moisture, monitoring cave drip and pool waters is desirable. Three major parameters that are basic to monitoring drip water are drip locations, drip rate, and drip volume. In addition to these basic parameters, drip water chemistry, microbiology, and temperature can be monitored in more complete programs. For pool waters, the basic parameter for monitoring is often water level. In more complete monitoring programs, pool water chemistry, microbiology, conductivity, and temperature would potentially be monitored.
Changes in the amount or distribution of dripping or pooling water in a cave may be the result of either natural processes (such as drought) or due to modifications caused by human action (changes in land use in the contributing watershed for the cave, changes in cave microclimate caused by management actions, etc.). Monitoring may be used to identify such changes and to plan mitigation, if appropriate. For example, monitoring of pool levels in Carlsbad Caverns (McLean, 1971, 1976) identified airflow through the elevator shaft as a significant impact on the cave and provided information that the park used to mitigate the impact.
Monitoring water quality (chemistry, microbiology, etc.) can provide important information on contaminants that may be brought into the cave and damage cave resources. Studies by Brooke (1996) and van der Heijde et al. (1997) identified areas of Carlsbad Caverns that had been impacted by contaminants brought into the cave from the surface (parking lots, sewage system, buildings, etc.) by infiltrating waters. Caldwell (1991) examined pools in Carlsbad Caverns to determine the impacts of visitors on their chemistry and microbiology.
Methods
As with cave meteorology, there are two basic approaches to monitoring drip and pool water parameters. The simplest approach is to manually monitor parameters with handheld instruments. Using automated sensors tied to data loggers is the higher-end approach.
Level 1 Methods
Manual measuring with handheld instrumentation. Monitoring the location and amount of dripping water in a cave is often accomplished manually. Several different methods can be used, depending on the type of monitoring, available resources, and accessibility of sites. Buecher (1999) and Sanz and Lopez (2000) discuss several manual drip monitoring methods. One approach is to monitor drip rate, drip volume and other parameters such as water chemistry at defined drip-monitoring locations. Drip rate can be estimated using a stopwatch, by either counting the number of drips in a set time or determining the time for a set number of drips. The estimate will improve as the observation time increases. Drip volume can be estimated by using a graduated cylinder to collect dripping water. Again, estimates can be made by determining either the volume in a particular time or the time required to fill to a particular volume. Water collected in such a study can be used to monitor drip water chemistry (Buecher, 1999; Musgrove and Banner, 2004). In cases where the drip rate of individual stalactites is highly variable, samples can be pooled to provide a smoothed rate of flow. This can be accomplished by using a larger bucket that collects drips from multiple stalactites or using a tarp to catch and channel flow from several stalactites. Another approach to monitoring the quantity of dripping water in a cave or cave area is to monitor water accumulation in random areas rather than choosing specific drips. Buecher (1999) did this by placing circular, 23 cm diameter pans in locations and measuring water accumulations over set times.
Specific conductivity (a measure of the electrical conduction of the water due to ions dissolved in it) is probably the most commonly measured chemical parameter in drip water monitoring. It is a preferred parameter both because it is relatively easy to measure using inexpensive handheld instruments and because it provides important information on the potential for the water to deposit or dissolve cave minerals.
Another very commonly measured water chemistry parameter (in dripping, pooling, and flowing water) is hydrogen ion activity (expressed as pH). The pH value of karst water is important in its own right; it is also used to calculate other values that provide important information about karst systems (such as saturation indices). Measuring pH can be problematic under field conditions, especially when small variations in pH can lead to large differences in calculated parameters. Sasowsky and Dalton (2005) provide a good discussion of the problems associated with field measurement of pH and provide recommendations for improving measurements.
Monitoring of pool water levels can be an important component of a monitoring program. Changes in pool level may indicate changes in cave climate. For example, changes in pool level at Carlsbad Caverns were an important indicator of increased evaporation due to the elevators (McLean, 1971, 1976). Monitoring pool water level can be accomplished manually by either measuring pool depth at a set point or measuring the distance to the pool surface from a set point.
Methods that take spot measurements of drip and pool parameters with handheld instruments can be accomplished quite cheaply. Commonly available instruments generally perform well for most cave situations. Trained volunteers or inexpensive personnel can perform the monitoring.
Level 2 Methods
Automatic measuring with datalogged instrumentation. Automated drip water monitoring is significantly more complex and uncommon (Genty and Deflandre, 1998). There are no standard, off-the-shelf solutions to monitoring drip frequency, drip volume, or drip chemistry. A variety of custom-built instruments have been installed for scientific research, but not for routine monitoring. Some of these instruments use a tipping bucket approach to measure frequency and volume of drips (Beddows et al., 2005). Less frequently, more exotic approaches, such as laser beams, photoelectric, or a vibrating drum principle (Baker and Brunsdon, 2003; Genty and Defl andre, 1998), are used. In general, automated monitoring of cave drips will require a person skilled in designing instruments.
Monitoring of pool water levels can also be automated. The most common means of automating measurement of pool levels is through the use of pressure transducers attached to data loggers. This method will generally require some level of specialized knowledge to set-up.
Pool or drip chemistry and/or microbiology. The monitoring of pool or drip chemistry depends on the parameters being measured. U.S. Geological Survey (variously dated) provides guidance on measuring water quality parameters. Krawczyk (1998) also describes procedures of measuring a variety of chemical parameters including pH, dissolved oxygen, specific conductivity, hardness, calcium, magnesium, sodium, potassium, bicarbonate, chlorides, sulfates, nitrates, phosphates, and silica. As with pool level, some of the water quality parameters can be monitored continuously or periodically with datalogged sensors. The ease and utility of this approach varies with parameters. For example, it is difficult to automate pH sensors for long-term monitoring, because accuracy, measurement drift, and calibration issues are significant in these sensors.
Microbiology can be an important parameter to monitor in infiltration water in either drips or pools. The most common reason for monitoring microbiology in cave drips and pools is to detect deleterious bacteria entering the cave due to contamination from human or animal waste. The presence of elevated coliform, fecal coliform, and E. coli are common indicators of potential contamination. These parameters are measured using standard procedures for other freshwater (including waste water and drinking water); Myers and Wilde (2003) provide guidance on standard, accepted procedures for biological indicators of water quality.
Sampling for pool or drip chemistry can generally be accomplished by volunteers or employees using inexpensive equipment and techniques. However, laboratories with specialized instruments are needed to measure many of the parameters. If such a lab is available for running analyses, these methods would generally be considered Level 2 methods. If, however, an onsite lab is going to be developed for this testing, the method would better be considered Level 3, due to the cost of equipment and skilled technicians needed to run analyses.
VITAL SIGN: MICROBIOLOGY
The study of the microbiology of caves is a relatively recent development, and it is evolving rapidly in terms of both methodologies and results. Recent studies have shown that microbes play an important role in a number of cave geological processes including wall corrosion and development of corrosion residues, precipitation of a variety of secondary minerals and speleothems, and sulfuric acid speleogenesis and cave enlargement (Barton
and Luiszer, 2005; Barton et al., 2001; Northup and Lavoie, 2001). The microbiology of caves can potentially be significantly altered by a large range of activities such as exploration, actions that lead to contamination of infiltrating water (land use changes, construction, etc.), and tour/caving activities (Hunter et al., 2004; Ikner et al., 2007). The potentially profound role that microbes play in cave processes and their susceptibility to alteration due to human activities make cave microbiology an important vital sign to monitor.
Methods
Level 3 Methods
Develop and implement specialized site-based monitoring. As noted above, cave microbiology is still in the relatively early stages of development as a science. Most of the studies that have been undertaken so far are exploratory in nature. They focus on what microbes are in the system, how different cave microbial ecosystems function, or how microbes are involved in different cave processes. For this reason, there are no standard methods that have been agreed upon for studying cave microbiological systems, let alone methods for standardized monitoring. Some parameters that are likely to be important in monitoring include microbe diversity, activity of various microbial ecosystem components, and the presence of microbial taxa indicating disturbance or perturbation of natural systems. Because of the interaction between surface and cave environments and the role of soil bacteria in the carbon dioxide fl ux in soils and groundwater, soil microbiology above the cave may also be an important indicator of changes and human impacts. Any microbiological monitoring, beyond simply identifying the presence of certain common bacteria associated with human activity (e.g., total coliform, fecal coliform, and E. coli) will require working with scientists using a variety of specialized laboratory techniques.
VITAL SIGN: STABILITY-BREAKDOWN, ROCKFALL AND PARTINGS
The stability of the cave ceiling in terms of number, location, size, and frequency of rockfalls can be an important vital sign to monitor. Breakdown in caves is a natural process that occurs for numerous reasons (E.L. White, 2005). The location, style, and cause of breakdowns vary spatially within the cave and temporally during the development of the cave. Monitoring of breakdown is generally undertaken for two somewhat different reasons. One purpose can be to understand the ongoing breakdown process to determine if some sort of mitigation is needed. A second purpose of monitoring breakdown may be for safety, i.e., determining when to limit people traveling under a particular area.
Monitoring breakdown to understand changes in the process due to changing cave conditions can be undertaken in several ways and at several time frames. However, monitoring for safety should provide some ability to predict the likelihood of additional breakdown. In addition, monitoring to understand the pattern of breakdown can be done on whatever time frame is convenient; that is, monitoring can take place annually, or on even longer time frames. Monitoring for human safety, on the other hand, should be done on time frames that are meaningful for protecting people from possible dangers. The more frequent the interval of monitoring, the better equipped you will be to warn people of potential dangers. The logical extension of this observation is that to have a true warning system for potential rockfall, real-time data gathering and analysis may be necessary.
Changes in rockfall number, location or size may indicate that changes have occurred which promote more rockfalls. For example, changes in entrance configuration that lead to more penetration in freezing temperatures can lead to increased rockfall due to freeze-thaw ice wedging.
Additional approaches to monitoring rockfall and slope stability may be found in the chapter on slope features and processes.
Methods
Level 1 Methods
Breakdown records. One of the simplest means to monitor breakdown is to simply record the date and location of each new piece of breakdown that occurs in a particular section of a cave. This is simple in theory, but it can be complex in practice. It may be difficult to distinguish new breakdown from existing breakdown in a cave passage with a rocky floor. In addition, if the cave is not visited very often, timing is impossible to determine and new rockfall may be hard to identify. This technique is probably most amenable to show caves where daily visitation occurs by people very familiar with the cave (guides); in addition, tour trails provide a clear area that can be monitored. This is an inexpensive method that can be accomplished with volunteer or other trained labor.
Manual crack monitoring. A simple, manual method to monitor movement on bedding plane partings and cracks that may lead to breakdown is to monitor the sizes of those cracks using mechanical means. One method is to place small wood or metal wedges into the cracks. If the wedges loosen, it may be an indication that movement is occurring on the cracks. A similar approach is to affix small strings or bands across the cracks. Breakage of the string or band can indicate movement. For this technique, bands or strings must be composed of a material that will not expand or contract signifi cantly under cave conditions. This technique will provide general information, but is not an adequate substitute for more precise methods that might be employed in conditions where signifi cant breakdown hazards are anticipated.
Level 3 Methods
Mining engineering stability techniques. To accurately monitor movement on cracks and bedding planes, technology and expertise is needed. Getting the appropriate assistance from a geological or mining engineer is especially important if the goal of monitoring is to increase safety. The mining industry uses a variety of techniques to monitor the stability of rock ceilings and walls. These include wire extensometers, EDM (electronic distance measurement) prism surveying, microseismic monitoring, surveying displacements, displacement transducers, time domain reflectometry, inclinometers, and LIDAR (Girard and McHugh, 2000; Bhatt and Mark, 2000). At Kartchner Caverns, wire line extensometers tied to a data logger were used to monitor several bedding planes during development at the cave.
VITAL SIGN: MINERAL GROWTH
Secondary mineral formations, or speleothems, are an important geological cave resource. The deposition and loss of these secondary minerals are very important processes. However, in most cases the processes are too slow to be monitored using simple processes. Seasonal mineral growths that may occur due to changes in cave microclimate represent an important group of exceptions to the general rule of the slow growth of cave formations. A variety of these types of seasonal mineral growths occur; most are related to seasonal changes in relative humidity. Seasonal drying leads to the growth of various minerals that are not stable under moist season cave conditions. Some examples include nitrocalcite at Kartchner Caverns and sulfates, such as gypsum, epsomite, and mirabilite in a number of caves including Mammoth Cave, Kentucky (Hill and Forti, 1997).
Color changes and staining of cave formations may be an indicator of human impacts that may be degrading the geological resources or the cave. In some cases local staining can be caused by cave visitors touching a formation. Groundwater contamination with sewage or metals may also result in changes in the color of formations in a particular area.
Methods
Level 1 Methods
Manual seasonal mineral presence mapping/inventory. Monitoring the seasonal growth and loss of cave mineral deposits is usually done manually. The extent of mineral deposits can be mapped in both space and time by trained individuals. The amount of training and equipment that is required to identify and map mineral deposits depends on the particular minerals that occur seasonally. Some seasonal minerals can be easily distinguished in the field, others require specialized mineralogical techniques. Often potential seasonal mineral deposits to be monitored are identified through mineralogical inventories. Following the initial identification of the minerals, visual examination may help to distinguish the mineral for seasonal mapping. Changes in the pattern and timing of mineral growth may indicate changes in regional climate or alterations to microclimate conditions in the cave. Because the deposition and disappearance of these minerals is seasonal, it is important to map the occurrences and extent of these minerals in different seasons.
Level 2 Methods
Photomonitoring and impact mapping/inventory. Color changes and staining of formations can be an important indicator of impacts to cave mineral resources. Monitoring for staining and color changes is probably best accomplished as part of a visitor impact mapping/inventory or photomonitoring program. These approaches are discussed in more detail in the Direct Visitor Impacts section above. To be able to accurately determine color and track changes, it is important to include a color standard in your photomonitoring pictures.
Level 3 Methods
LIDAR or time-of-flight laser scanning. As LIDAR and other laser scanning techniques have improved, it has become possible to measure the growth rates on some of the faster growing speleothems. In the near future it is possible that this technology may become widespread and inexpensive enough that it can be used for monitoring changes in speleothem growth rates. At this point, however, the technology is more appropriate for scientific studies of speleothem growth than it is for actually monitoring growth. This method involves expensive, specialized equipment which requires skilled operators. A more complete discussion of the limitations of this technique can be found in the Direct Visitor Impacts section above.
VITAL SIGN: SURFACE EXPRESSIONSAND PROCESSES
Although caves are an underground feature, they are intimately tied to the surface by conduits that transport water, sediment, and organic matter both in and out. These conduits vary from large entrances and springs to microscopic cracks and porosity. Karst processes closely link the surface to the caves through springs, sinkholes, cracks, etc.
Monitoring of the surface may provide important information about the state of the cave and karst resources. In addition, cave and karst resources can be degraded due to actions on the surface. For these reasons, monitoring of the surface can be an important component of cave and karst monitoring.
This discussion focuses on two major types of surface monitoring in karst. The first type of important surface monitoring in karst is monitoring springs for changes in water quality and quantity. The second is the use of remotely sensed data (photographs, satellite data, and airborne LIDAR) to monitor karst areas for changes (land use, sinkhole formation, etc.).
Springs are an important location for monitoring potential impacts on cave and karst systems. Changes in the quantity and quality of spring water may be symptomatic of changes that are degrading cave resources, such as changes to land use or contaminant leakage.
The topic of monitoring land use changes is largely beyond the scope of this book. However, land use changes may alter or degrade cave and karst resources by affecting water quantity, quality, or distribution. Monitoring land use changes is especially important for managers who have caves whose watersheds extend beyond the area they have control over. In cases such as these, actions of land managers many miles away may result in changes in caves that are being managed to protect resources. For example, in the well- eveloped karst of Missouri, dye tracing has indicated that water from as far as 61 km (38 miles) away is discharged at Big Spring (Imes and Fredrick, 2001).
Methods
Level 1–3 Methods
Spring water quality and quantity monitoring. Springs are often an important surface expression of karst processes. A detailed discussion of water flow and quality monitoring techniques is beyond the scope of this book. Nevertheless, monitoring water quantity and quality can be very important in monitoring the overall state of karst systems and related caves. As with other general water quality and quantity monitoring (such as in streams and wells), spring monitoring programs should be designed in consultation with hydrologists or hydrogeologists who specialize in karst aquifers. W.B. White (2005) provides insight into spring processes and the ways spring discharge and chemistry can be monitored. The techniques and approaches for monitoring spring flow and water quality vary depending on the needs and available resources. Some techniques can be done inexpensively using volunteers; others require scientists, complex equipment, and specialized laboratory analyses.
Level 3 Methods
Remote sensing of karst landscape changes. Identifying changes in landscape (such as development of new sinkholes inkarst) and monitoring changes in land use that may affect cave and karst resources is often best achieved using periodic remote sensing methods such as airborne and satellite imagery and data gathering. In addition to visible spectrum imagery, several types of sensing using other portions of the electromagnetic spectrum have been used to study karst processes and terrains.
Airborne LIDAR has been used to identify karst features in a few studies. Notably, it has been used to map karst topography in rain forests in Alaska (Baichtel and Langendoen, 2001; Langendoen and Baichtel, 2004). It has also been used in the low-lying karst of central Florida to both identify and map sinkholes (Seale et. al., 2004) and also to predict the location of potentially hazardous subsurface features (Montane and Whitman, 2000). These studies have focused on the use of airborne LIDAR to inventory karst features rather than monitoring them for changes; however, monitoring would be an additional possibility once inventory was completed. Airborne LIDAR is a powerful tool for monitoring changes in karst landscapes. It is also an expensive tool that requires specialized equipment and personnel.
Remotely sensed thermal infrared imagery can also prove useful in identifying and potentially monitoring karst features. Campbell et al. (1996) successfully used airborne thermography to map springs, caves, swallets, and losing stream reaches in northern Alabama (Campbell et al., 1996; Campbell and Keith, 2001) and Tennessee (Campbell and Singer, 2001). The use of thermography to identify or monitor non-spring cave entrances is less developed, but it is an area of active research (Thompson and Marvin, 2006).
Remote sensing will generally require participation of experienced scientists. If the remote sensing is being accomplished specifically for karst monitoring purposes, it will also require mobilization of expensive, specialized equipment (such as tasking airplanes and satellites). In addition, the data processing requirements are substantial. The use of existing, off-the-shelf, remotely sensed, georeferenced data sets will reduce the costs for data acquisition; however, specialized knowledge will likely still be needed to interpret the data.
VITAL SIGN: REGIONAL GROUNDWATER LEVELS AND QUANTITY
Caves, and solutional caves in particular, are often an expression of the regional groundwater flow. Changes in local or regional aquifers may affect cave resources by changing cave microclimate, flooding previously dry areas, drying existing pools or streams, or altering dissolution or deposition of minerals. Both natural processes (such as drought) and human processes (including groundwater pumping, surface flow alteration, and chemical contamination) can alter the water quantity and quality of local and regional aquifers. Processes, such as pumping or drought, which lower water levels in regional aquifers, may impact caves in the area. In addition, these processes may cause other impacts in karst terrain; one notable impact is causing or increasing sinkhole collapse.
Monitoring local or regional karst aquifers associated with a cave may be an important tool for understanding and protecting cave resources of all types. Regional groundwater can be monitored in caves (e.g., pool water and stream water) or on the surface using springs. Methods for monitoring these vital signs are discussed elsewhere in the manual. In addition, wells into karst aquifers may be used to monitor groundwater levels and chemistry. A variety of agencies can assist in developing appropriate water quality monitoring programs.
Methods
Level 1 Methods
Spot monitoring of wells. Wells for monitoring water quality and quantity in karst aquifers may be specifically constructed for the purpose, or may be preexisting. The cost and expertise to develop this kind of monitoring depends greatly on whether existing wells can be used or whether new wells must be drilled for this purpose. Static (unpumped) wells are preferred for monitoring water levels in an aquifer. Having a pump in the well (whether it is used at other times or not) greatly aids water sampling for water quality monitoring.
The water level and quality in these wells can be monitored either periodically (as at Kartchner Caverns, Graf, 1999; Toomey, 2005; Toomey and Nolan, 2005) or continuously (as is done in many aquifers that provide significant drinking water like the Edwards aquifer in Texas). Manually measuring the water level in wells, or more accurately measuring the depth to the top of the water, is easily accomplished by three common methods: tape or cord, electrical sounder, or air line (Powell and Rogers, 2005; Thornhill 1989). In tape (or cord) and electrical sounder methods, a measuring device is lowered into the well until it hits water (as indicated by marking on the tape or completing an electrical circuit). In air line methods, a known length of thin air tubing is placed into the well. The length must be longer than the depth to water. The amount of air pressure needed to purge the line of air provides a measure of the length of tube that contains water. That length is subtracted from the total length of tube installed to indicate depth to water (Powell and Rogers, 2005). The frequency of measuring depends on the dynamics of the aquifer and the nature of potential threats from changing aquifer levels. In relatively nondynamic aquifers that are subject to mainly natural changes, such as at Kartchner Caverns State Park, Arizona, monthly monitoring may be suffi cient to understand trends (Toomey, 2005; Toomey and Nolan, 2005) in groundwater level and their relationship to cave changes (Fig. 8). If, however, groundwater pumping is a significant issue that may affect cave resources, more frequent monitoring may be necessary. These monitoring methods require relatively little training or specialized equipment.
Level 2 Methods
Monitoring of well chemistry. Water quality measurements depend on potential threats to the regional and local aquifer and caves. Basic parameters for monitoring karst waters include temperature, pH, dissolved oxygen, specific conductivity, hardness, calcium, magnesium, sodium, potassium, bicarbonate, chlorides, sulfates, nitrates, phosphates, and silica (U.S. Geological Survey, variously dated; Krawczyk, 1998). In addition, microbiological parameters, such as coliform bacteria, fecal coliform bacteria, and E. coli may be important parameters for detecting contamination from septic systems. Depending on land use in the watershed of interest, it may also be important to monitor for a variety of organic and inorganic pollutants. Collecting samples for groundwater chemistry is fairly straightforward and inexpensive. However, many chemical analyses require specialized laboratory equipment.
Automatic monitoring of groundwater levels in wells. Continuous monitoring of groundwater levels is achieved by attaching a sensor that measures water depth in the well to a data logger, which records the level at set intervals. Two different kinds of sensors are commonly used for measuring water depth in wells. The first kind consists of floats that measure the elevation of the top of the water. The second kind consists of pressure transducers that measure the height of the water column over the transducer. Automatic monitoring of groundwater levels requires specialized sensors and some technical knowledge to install them.
Photomonitoring. Repeat photography is another method for monitoring ice extent, although with very clear ice, it may be difficult to discern ice extent in photographs. Historical photographs may be used to estimate past ice volumes and extend the monitoring record (Luetscher, et al., 2005). Werker and Hildreth-Werker (1996) and Hildreth-Werker (2006) provide many useful suggestions for improving photomonitoring of the cave environment.
Level 3 Methods
LIDAR or time-of-fl ight laser scanning. Repeat laser scanning can track ice extent accurately; however, the technique requires expensive equipment and highly trained specialists for data acquisition and reduction. A more complete discussion of
the limitations of this technique can be found in the Direct Visitor Impacts section above.
VITAL SIGN: CAVE DRIP AND POOL WATER
Dripping and pooled water are important components of many cave environments. Dripping water transports minerals, microbes, and chemicals into the cave. It is responsible for depositing minerals, resulting in the growth of many types of cave formations, most notably stalactites and stalagmites (Hill and Forti, 1997). Pooled water formed by infiltration (through dripping or flowing) is also responsible for the formation of several types of formations, such as shelfstone, rafts, pool fingers, and pool spar (Hill and Forti, 1997). Dripping and pooled water also acts to maintain cave moisture. Drip water can also transport contaminants into caves.
Because of its crucial roles in depositing cave formations and maintaining moisture, monitoring cave drip and pool waters is desirable. Three major parameters that are basic to monitoring drip water are drip locations, drip rate, and drip volume. In addition to these basic parameters, drip water chemistry, microbiology, and temperature can be monitored in more complete programs. For pool waters, the basic parameter for monitoring is often water level. In more complete monitoring programs, pool water chemistry, microbiology, conductivity, and temperature would potentially be monitored.
Changes in the amount or distribution of dripping or pooling water in a cave may be the result of either natural processes (such as drought) or due to modifications caused by human action (changes in land use in the contributing watershed for the cave, changes in cave microclimate caused by management actions, etc.). Monitoring may be used to identify such changes and to plan mitigation, if appropriate. For example, monitoring of pool levels in Carlsbad Caverns (McLean, 1971, 1976) identified airflow through the elevator shaft as a significant impact on the cave and provided information that the park used to mitigate the impact.
Monitoring water quality (chemistry, microbiology, etc.) can provide important information on contaminants that may be brought into the cave and damage cave resources. Studies by Brooke (1996) and van der Heijde et al. (1997) identified areas of Carlsbad Caverns that had been impacted by contaminants brought into the cave from the surface (parking lots, sewage system, buildings, etc.) by infiltrating waters. Caldwell (1991) examined pools in Carlsbad Caverns to determine the impacts of visitors on their chemistry and microbiology.
Methods
As with cave meteorology, there are two basic approaches to monitoring drip and pool water parameters. The simplest approach is to manually monitor parameters with handheld instruments. Using automated sensors tied to data loggers is the higher-end approach.
Level 1 Methods
Manual measuring with handheld instrumentation. Monitoring the location and amount of dripping water in a cave is often accomplished manually. Several different methods can be used, depending on the type of monitoring, available resources, and accessibility of sites. Buecher (1999) and Sanz and Lopez (2000) discuss several manual drip monitoring methods. One approach is to monitor drip rate, drip volume and other parameters such as water chemistry at defined drip-monitoring locations. Drip rate can be estimated using a stopwatch, by either counting the number of drips in a set time or determining the time for a set number of drips. The estimate will improve as the observation time increases. Drip volume can be estimated by using a graduated cylinder to collect dripping water. Again, estimates can be made by determining either the volume in a particular time or the time required to fill to a particular volume. Water collected in such a study can be used to monitor drip water chemistry (Buecher, 1999; Musgrove and Banner, 2004). In cases where the drip rate of individual stalactites is highly variable, samples can be pooled to provide a smoothed rate of flow. This can be accomplished by using a larger bucket that collects drips from multiple stalactites or using a tarp to catch and channel flow from several stalactites. Another approach to monitoring the quantity of dripping water in a cave or cave area is to monitor water accumulation in random areas rather than choosing specific drips. Buecher (1999) did this by placing circular, 23 cm diameter pans in locations and measuring water accumulations over set times.
Specific conductivity (a measure of the electrical conduction of the water due to ions dissolved in it) is probably the most commonly measured chemical parameter in drip water monitoring. It is a preferred parameter both because it is relatively easy to measure using inexpensive handheld instruments and because it provides important information on the potential for the water to deposit or dissolve cave minerals.
Another very commonly measured water chemistry parameter (in dripping, pooling, and flowing water) is hydrogen ion activity (expressed as pH). The pH value of karst water is important in its own right; it is also used to calculate other values that provide important information about karst systems (such as saturation indices). Measuring pH can be problematic under field conditions, especially when small variations in pH can lead to large differences in calculated parameters. Sasowsky and Dalton (2005) provide a good discussion of the problems associated with field measurement of pH and provide recommendations for improving measurements.
Monitoring of pool water levels can be an important component of a monitoring program. Changes in pool level may indicate changes in cave climate. For example, changes in pool level at Carlsbad Caverns were an important indicator of increased evaporation due to the elevators (McLean, 1971, 1976). Monitoring pool water level can be accomplished manually by either measuring pool depth at a set point or measuring the distance to the pool surface from a set point.
Methods that take spot measurements of drip and pool parameters with handheld instruments can be accomplished quite cheaply. Commonly available instruments generally perform well for most cave situations. Trained volunteers or inexpensive personnel can perform the monitoring.
Level 2 Methods
Automatic measuring with datalogged instrumentation. Automated drip water monitoring is significantly more complex and uncommon (Genty and Deflandre, 1998). There are no standard, off-the-shelf solutions to monitoring drip frequency, drip volume, or drip chemistry. A variety of custom-built instruments have been installed for scientific research, but not for routine monitoring. Some of these instruments use a tipping bucket approach to measure frequency and volume of drips (Beddows et al., 2005). Less frequently, more exotic approaches, such as laser beams, photoelectric, or a vibrating drum principle (Baker and Brunsdon, 2003; Genty and Defl andre, 1998), are used. In general, automated monitoring of cave drips will require a person skilled in designing instruments.
Monitoring of pool water levels can also be automated. The most common means of automating measurement of pool levels is through the use of pressure transducers attached to data loggers. This method will generally require some level of specialized knowledge to set-up.
Pool or drip chemistry and/or microbiology. The monitoring of pool or drip chemistry depends on the parameters being measured. U.S. Geological Survey (variously dated) provides guidance on measuring water quality parameters. Krawczyk (1998) also describes procedures of measuring a variety of chemical parameters including pH, dissolved oxygen, specific conductivity, hardness, calcium, magnesium, sodium, potassium, bicarbonate, chlorides, sulfates, nitrates, phosphates, and silica. As with pool level, some of the water quality parameters can be monitored continuously or periodically with datalogged sensors. The ease and utility of this approach varies with parameters. For example, it is difficult to automate pH sensors for long-term monitoring, because accuracy, measurement drift, and calibration issues are significant in these sensors.
Microbiology can be an important parameter to monitor in infiltration water in either drips or pools. The most common reason for monitoring microbiology in cave drips and pools is to detect deleterious bacteria entering the cave due to contamination from human or animal waste. The presence of elevated coliform, fecal coliform, and E. coli are common indicators of potential contamination. These parameters are measured using standard procedures for other freshwater (including waste water and drinking water); Myers and Wilde (2003) provide guidance on standard, accepted procedures for biological indicators of water quality.
Sampling for pool or drip chemistry can generally be accomplished by volunteers or employees using inexpensive equipment and techniques. However, laboratories with specialized instruments are needed to measure many of the parameters. If such a lab is available for running analyses, these methods would generally be considered Level 2 methods. If, however, an onsite lab is going to be developed for this testing, the method would better be considered Level 3, due to the cost of equipment and skilled technicians needed to run analyses.
VITAL SIGN: MICROBIOLOGY
The study of the microbiology of caves is a relatively recent development, and it is evolving rapidly in terms of both methodologies and results. Recent studies have shown that microbes play an important role in a number of cave geological processes including wall corrosion and development of corrosion residues, precipitation of a variety of secondary minerals and speleothems, and sulfuric acid speleogenesis and cave enlargement (Barton
and Luiszer, 2005; Barton et al., 2001; Northup and Lavoie, 2001). The microbiology of caves can potentially be significantly altered by a large range of activities such as exploration, actions that lead to contamination of infiltrating water (land use changes, construction, etc.), and tour/caving activities (Hunter et al., 2004; Ikner et al., 2007). The potentially profound role that microbes play in cave processes and their susceptibility to alteration due to human activities make cave microbiology an important vital sign to monitor.
Methods
Level 3 Methods
Develop and implement specialized site-based monitoring. As noted above, cave microbiology is still in the relatively early stages of development as a science. Most of the studies that have been undertaken so far are exploratory in nature. They focus on what microbes are in the system, how different cave microbial ecosystems function, or how microbes are involved in different cave processes. For this reason, there are no standard methods that have been agreed upon for studying cave microbiological systems, let alone methods for standardized monitoring. Some parameters that are likely to be important in monitoring include microbe diversity, activity of various microbial ecosystem components, and the presence of microbial taxa indicating disturbance or perturbation of natural systems. Because of the interaction between surface and cave environments and the role of soil bacteria in the carbon dioxide fl ux in soils and groundwater, soil microbiology above the cave may also be an important indicator of changes and human impacts. Any microbiological monitoring, beyond simply identifying the presence of certain common bacteria associated with human activity (e.g., total coliform, fecal coliform, and E. coli) will require working with scientists using a variety of specialized laboratory techniques.
VITAL SIGN: STABILITY-BREAKDOWN, ROCKFALL AND PARTINGS
The stability of the cave ceiling in terms of number, location, size, and frequency of rockfalls can be an important vital sign to monitor. Breakdown in caves is a natural process that occurs for numerous reasons (E.L. White, 2005). The location, style, and cause of breakdowns vary spatially within the cave and temporally during the development of the cave. Monitoring of breakdown is generally undertaken for two somewhat different reasons. One purpose can be to understand the ongoing breakdown process to determine if some sort of mitigation is needed. A second purpose of monitoring breakdown may be for safety, i.e., determining when to limit people traveling under a particular area.
Monitoring breakdown to understand changes in the process due to changing cave conditions can be undertaken in several ways and at several time frames. However, monitoring for safety should provide some ability to predict the likelihood of additional breakdown. In addition, monitoring to understand the pattern of breakdown can be done on whatever time frame is convenient; that is, monitoring can take place annually, or on even longer time frames. Monitoring for human safety, on the other hand, should be done on time frames that are meaningful for protecting people from possible dangers. The more frequent the interval of monitoring, the better equipped you will be to warn people of potential dangers. The logical extension of this observation is that to have a true warning system for potential rockfall, real-time data gathering and analysis may be necessary.
Changes in rockfall number, location or size may indicate that changes have occurred which promote more rockfalls. For example, changes in entrance configuration that lead to more penetration in freezing temperatures can lead to increased rockfall due to freeze-thaw ice wedging.
Additional approaches to monitoring rockfall and slope stability may be found in the chapter on slope features and processes.
Methods
Level 1 Methods
Breakdown records. One of the simplest means to monitor breakdown is to simply record the date and location of each new piece of breakdown that occurs in a particular section of a cave. This is simple in theory, but it can be complex in practice. It may be difficult to distinguish new breakdown from existing breakdown in a cave passage with a rocky floor. In addition, if the cave is not visited very often, timing is impossible to determine and new rockfall may be hard to identify. This technique is probably most amenable to show caves where daily visitation occurs by people very familiar with the cave (guides); in addition, tour trails provide a clear area that can be monitored. This is an inexpensive method that can be accomplished with volunteer or other trained labor.
Manual crack monitoring. A simple, manual method to monitor movement on bedding plane partings and cracks that may lead to breakdown is to monitor the sizes of those cracks using mechanical means. One method is to place small wood or metal wedges into the cracks. If the wedges loosen, it may be an indication that movement is occurring on the cracks. A similar approach is to affix small strings or bands across the cracks. Breakage of the string or band can indicate movement. For this technique, bands or strings must be composed of a material that will not expand or contract signifi cantly under cave conditions. This technique will provide general information, but is not an adequate substitute for more precise methods that might be employed in conditions where signifi cant breakdown hazards are anticipated.
Level 3 Methods
Mining engineering stability techniques. To accurately monitor movement on cracks and bedding planes, technology and expertise is needed. Getting the appropriate assistance from a geological or mining engineer is especially important if the goal of monitoring is to increase safety. The mining industry uses a variety of techniques to monitor the stability of rock ceilings and walls. These include wire extensometers, EDM (electronic distance measurement) prism surveying, microseismic monitoring, surveying displacements, displacement transducers, time domain reflectometry, inclinometers, and LIDAR (Girard and McHugh, 2000; Bhatt and Mark, 2000). At Kartchner Caverns, wire line extensometers tied to a data logger were used to monitor several bedding planes during development at the cave.
VITAL SIGN: MINERAL GROWTH
Secondary mineral formations, or speleothems, are an important geological cave resource. The deposition and loss of these secondary minerals are very important processes. However, in most cases the processes are too slow to be monitored using simple processes. Seasonal mineral growths that may occur due to changes in cave microclimate represent an important group of exceptions to the general rule of the slow growth of cave formations. A variety of these types of seasonal mineral growths occur; most are related to seasonal changes in relative humidity. Seasonal drying leads to the growth of various minerals that are not stable under moist season cave conditions. Some examples include nitrocalcite at Kartchner Caverns and sulfates, such as gypsum, epsomite, and mirabilite in a number of caves including Mammoth Cave, Kentucky (Hill and Forti, 1997).
Color changes and staining of cave formations may be an indicator of human impacts that may be degrading the geological resources or the cave. In some cases local staining can be caused by cave visitors touching a formation. Groundwater contamination with sewage or metals may also result in changes in the color of formations in a particular area.
Methods
Level 1 Methods
Manual seasonal mineral presence mapping/inventory. Monitoring the seasonal growth and loss of cave mineral deposits is usually done manually. The extent of mineral deposits can be mapped in both space and time by trained individuals. The amount of training and equipment that is required to identify and map mineral deposits depends on the particular minerals that occur seasonally. Some seasonal minerals can be easily distinguished in the field, others require specialized mineralogical techniques. Often potential seasonal mineral deposits to be monitored are identified through mineralogical inventories. Following the initial identification of the minerals, visual examination may help to distinguish the mineral for seasonal mapping. Changes in the pattern and timing of mineral growth may indicate changes in regional climate or alterations to microclimate conditions in the cave. Because the deposition and disappearance of these minerals is seasonal, it is important to map the occurrences and extent of these minerals in different seasons.
Level 2 Methods
Photomonitoring and impact mapping/inventory. Color changes and staining of formations can be an important indicator of impacts to cave mineral resources. Monitoring for staining and color changes is probably best accomplished as part of a visitor impact mapping/inventory or photomonitoring program. These approaches are discussed in more detail in the Direct Visitor Impacts section above. To be able to accurately determine color and track changes, it is important to include a color standard in your photomonitoring pictures.
Level 3 Methods
LIDAR or time-of-flight laser scanning. As LIDAR and other laser scanning techniques have improved, it has become possible to measure the growth rates on some of the faster growing speleothems. In the near future it is possible that this technology may become widespread and inexpensive enough that it can be used for monitoring changes in speleothem growth rates. At this point, however, the technology is more appropriate for scientific studies of speleothem growth than it is for actually monitoring growth. This method involves expensive, specialized equipment which requires skilled operators. A more complete discussion of the limitations of this technique can be found in the Direct Visitor Impacts section above.
VITAL SIGN: SURFACE EXPRESSIONSAND PROCESSES
Although caves are an underground feature, they are intimately tied to the surface by conduits that transport water, sediment, and organic matter both in and out. These conduits vary from large entrances and springs to microscopic cracks and porosity. Karst processes closely link the surface to the caves through springs, sinkholes, cracks, etc.
Monitoring of the surface may provide important information about the state of the cave and karst resources. In addition, cave and karst resources can be degraded due to actions on the surface. For these reasons, monitoring of the surface can be an important component of cave and karst monitoring.
This discussion focuses on two major types of surface monitoring in karst. The first type of important surface monitoring in karst is monitoring springs for changes in water quality and quantity. The second is the use of remotely sensed data (photographs, satellite data, and airborne LIDAR) to monitor karst areas for changes (land use, sinkhole formation, etc.).
Springs are an important location for monitoring potential impacts on cave and karst systems. Changes in the quantity and quality of spring water may be symptomatic of changes that are degrading cave resources, such as changes to land use or contaminant leakage.
The topic of monitoring land use changes is largely beyond the scope of this book. However, land use changes may alter or degrade cave and karst resources by affecting water quantity, quality, or distribution. Monitoring land use changes is especially important for managers who have caves whose watersheds extend beyond the area they have control over. In cases such as these, actions of land managers many miles away may result in changes in caves that are being managed to protect resources. For example, in the well- eveloped karst of Missouri, dye tracing has indicated that water from as far as 61 km (38 miles) away is discharged at Big Spring (Imes and Fredrick, 2001).
Methods
Level 1–3 Methods
Spring water quality and quantity monitoring. Springs are often an important surface expression of karst processes. A detailed discussion of water flow and quality monitoring techniques is beyond the scope of this book. Nevertheless, monitoring water quantity and quality can be very important in monitoring the overall state of karst systems and related caves. As with other general water quality and quantity monitoring (such as in streams and wells), spring monitoring programs should be designed in consultation with hydrologists or hydrogeologists who specialize in karst aquifers. W.B. White (2005) provides insight into spring processes and the ways spring discharge and chemistry can be monitored. The techniques and approaches for monitoring spring flow and water quality vary depending on the needs and available resources. Some techniques can be done inexpensively using volunteers; others require scientists, complex equipment, and specialized laboratory analyses.
Level 3 Methods
Remote sensing of karst landscape changes. Identifying changes in landscape (such as development of new sinkholes inkarst) and monitoring changes in land use that may affect cave and karst resources is often best achieved using periodic remote sensing methods such as airborne and satellite imagery and data gathering. In addition to visible spectrum imagery, several types of sensing using other portions of the electromagnetic spectrum have been used to study karst processes and terrains.
Airborne LIDAR has been used to identify karst features in a few studies. Notably, it has been used to map karst topography in rain forests in Alaska (Baichtel and Langendoen, 2001; Langendoen and Baichtel, 2004). It has also been used in the low-lying karst of central Florida to both identify and map sinkholes (Seale et. al., 2004) and also to predict the location of potentially hazardous subsurface features (Montane and Whitman, 2000). These studies have focused on the use of airborne LIDAR to inventory karst features rather than monitoring them for changes; however, monitoring would be an additional possibility once inventory was completed. Airborne LIDAR is a powerful tool for monitoring changes in karst landscapes. It is also an expensive tool that requires specialized equipment and personnel.
Remotely sensed thermal infrared imagery can also prove useful in identifying and potentially monitoring karst features. Campbell et al. (1996) successfully used airborne thermography to map springs, caves, swallets, and losing stream reaches in northern Alabama (Campbell et al., 1996; Campbell and Keith, 2001) and Tennessee (Campbell and Singer, 2001). The use of thermography to identify or monitor non-spring cave entrances is less developed, but it is an area of active research (Thompson and Marvin, 2006).
Remote sensing will generally require participation of experienced scientists. If the remote sensing is being accomplished specifically for karst monitoring purposes, it will also require mobilization of expensive, specialized equipment (such as tasking airplanes and satellites). In addition, the data processing requirements are substantial. The use of existing, off-the-shelf, remotely sensed, georeferenced data sets will reduce the costs for data acquisition; however, specialized knowledge will likely still be needed to interpret the data.
VITAL SIGN: REGIONAL GROUNDWATER LEVELS AND QUANTITY
Caves, and solutional caves in particular, are often an expression of the regional groundwater flow. Changes in local or regional aquifers may affect cave resources by changing cave microclimate, flooding previously dry areas, drying existing pools or streams, or altering dissolution or deposition of minerals. Both natural processes (such as drought) and human processes (including groundwater pumping, surface flow alteration, and chemical contamination) can alter the water quantity and quality of local and regional aquifers. Processes, such as pumping or drought, which lower water levels in regional aquifers, may impact caves in the area. In addition, these processes may cause other impacts in karst terrain; one notable impact is causing or increasing sinkhole collapse.
Monitoring local or regional karst aquifers associated with a cave may be an important tool for understanding and protecting cave resources of all types. Regional groundwater can be monitored in caves (e.g., pool water and stream water) or on the surface using springs. Methods for monitoring these vital signs are discussed elsewhere in the manual. In addition, wells into karst aquifers may be used to monitor groundwater levels and chemistry. A variety of agencies can assist in developing appropriate water quality monitoring programs.
Methods
Level 1 Methods
Spot monitoring of wells. Wells for monitoring water quality and quantity in karst aquifers may be specifically constructed for the purpose, or may be preexisting. The cost and expertise to develop this kind of monitoring depends greatly on whether existing wells can be used or whether new wells must be drilled for this purpose. Static (unpumped) wells are preferred for monitoring water levels in an aquifer. Having a pump in the well (whether it is used at other times or not) greatly aids water sampling for water quality monitoring.
The water level and quality in these wells can be monitored either periodically (as at Kartchner Caverns, Graf, 1999; Toomey, 2005; Toomey and Nolan, 2005) or continuously (as is done in many aquifers that provide significant drinking water like the Edwards aquifer in Texas). Manually measuring the water level in wells, or more accurately measuring the depth to the top of the water, is easily accomplished by three common methods: tape or cord, electrical sounder, or air line (Powell and Rogers, 2005; Thornhill 1989). In tape (or cord) and electrical sounder methods, a measuring device is lowered into the well until it hits water (as indicated by marking on the tape or completing an electrical circuit). In air line methods, a known length of thin air tubing is placed into the well. The length must be longer than the depth to water. The amount of air pressure needed to purge the line of air provides a measure of the length of tube that contains water. That length is subtracted from the total length of tube installed to indicate depth to water (Powell and Rogers, 2005). The frequency of measuring depends on the dynamics of the aquifer and the nature of potential threats from changing aquifer levels. In relatively nondynamic aquifers that are subject to mainly natural changes, such as at Kartchner Caverns State Park, Arizona, monthly monitoring may be suffi cient to understand trends (Toomey, 2005; Toomey and Nolan, 2005) in groundwater level and their relationship to cave changes (Fig. 8). If, however, groundwater pumping is a significant issue that may affect cave resources, more frequent monitoring may be necessary. These monitoring methods require relatively little training or specialized equipment.
Level 2 Methods
Monitoring of well chemistry. Water quality measurements depend on potential threats to the regional and local aquifer and caves. Basic parameters for monitoring karst waters include temperature, pH, dissolved oxygen, specific conductivity, hardness, calcium, magnesium, sodium, potassium, bicarbonate, chlorides, sulfates, nitrates, phosphates, and silica (U.S. Geological Survey, variously dated; Krawczyk, 1998). In addition, microbiological parameters, such as coliform bacteria, fecal coliform bacteria, and E. coli may be important parameters for detecting contamination from septic systems. Depending on land use in the watershed of interest, it may also be important to monitor for a variety of organic and inorganic pollutants. Collecting samples for groundwater chemistry is fairly straightforward and inexpensive. However, many chemical analyses require specialized laboratory equipment.
Automatic monitoring of groundwater levels in wells. Continuous monitoring of groundwater levels is achieved by attaching a sensor that measures water depth in the well to a data logger, which records the level at set intervals. Two different kinds of sensors are commonly used for measuring water depth in wells. The first kind consists of floats that measure the elevation of the top of the water. The second kind consists of pressure transducers that measure the height of the water column over the transducer. Automatic monitoring of groundwater levels requires specialized sensors and some technical knowledge to install them.
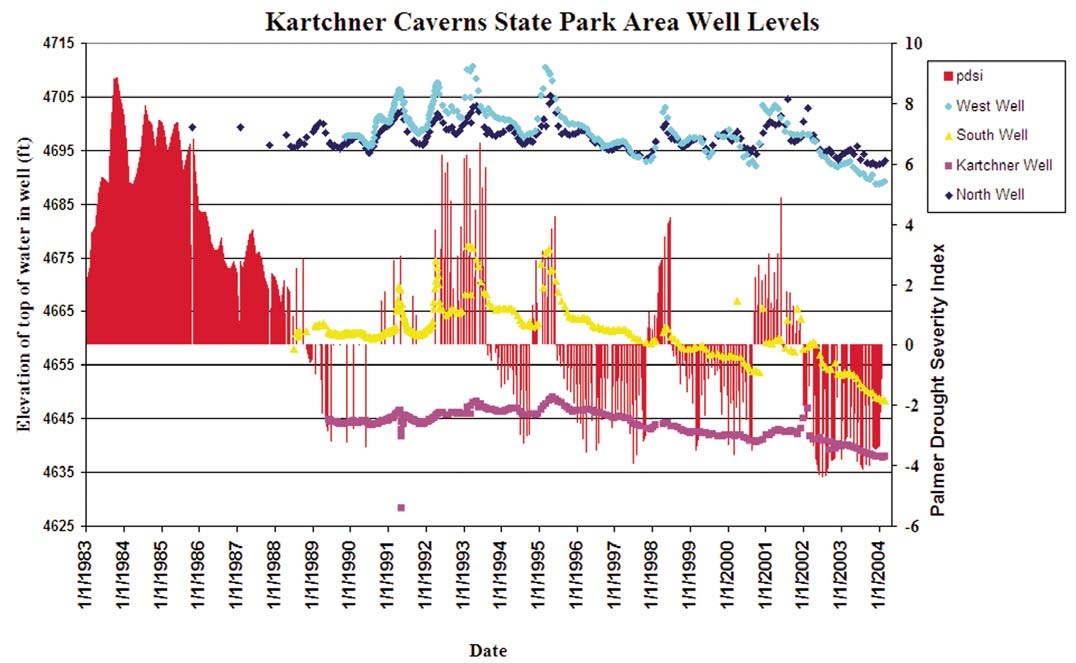
VITAL SIGN: FLUVIAL PROCESSES
In many caves, particularly those in karst landscapes, water flows in underground streams and rivers. Those streams and rivers are important for many reasons. They are often the main agent of ongoing speleogenesis in their caves. They provide important habitats for numerous aquatic species (including numerous endangered ones). The water in karst streams and rivers is often the groundwater used for drinking water.
The properties of these streams and rivers, including water quality, water quantity, sediment characteristics, and distribution of water inputs, are subject to alterations that may degrade cave and karst systems. Water quality can be affected by human activities and land use changes (septic systems, non-point-source pollution, and conversion to or from agriculture). The quantity and distribution of waters can be impacted by such things as impervious cover, channelization, sinkhole modification, and storm water diversion. Changing land use patterns around sinkholesand other inputs can radically change the sediment load, balance,
and characteristics of a cave stream. Changes such as these can, in turn, alter the bed characteristics.
Methods
Monitoring of fluvial processes is covered in a separate chapter in this manual. Many of the approaches described in that chapter can also be applied in cave streams. Among the most important parameters for monitoring cave streams are water quantity, turbidity, and water chemical characteristics. Changes in stream bed, in particular siltation or scouring, may also be quite important; however, these may have more effects on biological systems in caves than on the geological resources themselves. In addition, various agencies, including National Park Service Water Resources Division, U.S. Geological Survey Water Resources Program, and the Environmental Protection Agency Office of Water can provide additional guidance and assistance in monitoring cave stream parameters. In addition, references such as U.S. Geological Survey (variously dated) and Krawczyk (1998) provide details on techniques that can be used.
Developing a monitoring program for a cave, set of caves, or caves and associated karst landscapes is a complex task involving many disciplines. Although this chapter has focused on vital signs that are either geological in nature or which may significantly influence geological processes and resources, a complete monitoring program would also focus on biological resources (including bats, other vertebrates, invertebrates, and microbiology), cultural resources (archaeological and historic), and paleontological resources. In addition, monitoring programs must follow a systematic inventory, which is necessary to identify the resources to be monitored and the potential impacts to them.
The Carlsbad Caverns National Park Cave and Karst Management Plan Environmental Assessment contains a well-developed cave monitoring plan (Carlsbad Caverns National Park, 2006). It discusses the existing monitoring at the park, the rationale used to decide whether new or additional monitoring should take place, and the procedures to be followed in developing new monitoring projects. It provides an excellent guidance for developing cave monitoring projects.
Since monitoring is presumably tied to vulnerable resources, management actions, and needs, it is important to identify the vital signs that are most subject to human impact under existing and potential conditions. The monitoring of some vital signs is likely to be important under a wide variety of conditions. For example, most cave monitoring plans will probably include at least some monitoring of cave microclimate. The reason for this is that cave microclimate may affect a wide range of geological, biological, and cultural resources in a cave. Other types of monitoring may be limited to caves that undergo particular impacts. For example, monitoring of lamp fl ora growth or lint deposition would probably be applicable only in show caves. Water quality monitoring is likely to be more important where a significant threat of contamination exists due to land use or human use of the cave.
Budget is also a significant factor when designing a monitoring program. Fortunately, most of the basic means for monitoring geologic resources in caves do not require expensive, specialized equipment, but they often require either trained or specialized personnel (Table 1). Automating some of the measuring, where possible, may reduce the time requirement for personnel. However, the larger volumes of data from this approach may require personnel with more training to adequately analyze the results.
At few, if any, caves has a monitoring plan been implemented that addresses the entire list of vital signs discussed in this chapter, let alone the biological, microbiological, paleontological, and cultural resource monitoring that would constitute a complete monitoring program. However, this list of vital signs should serve as a guide to what might be monitored, depending on resources needing management, potential and actual threats, budget, and availability of experienced assistance.
This chapter benefited significantly from countless conversations between the author and Rick Olson, Ginger Nolan, Robert Casavant, Douglas Graeme, Johnathan Jernigan, Nick Crawford, Chris Groves, Penny Boston, Warren Campbell, Arrigo Cigna, and a variety of other cave resource managers. Rick Olson, Chuck Bitting, Ron Karpilo, and especially John Roth reviewed the manuscript and thus substantially improved it. Any errors of omission or commission, however, are mine.
In many caves, particularly those in karst landscapes, water flows in underground streams and rivers. Those streams and rivers are important for many reasons. They are often the main agent of ongoing speleogenesis in their caves. They provide important habitats for numerous aquatic species (including numerous endangered ones). The water in karst streams and rivers is often the groundwater used for drinking water.
The properties of these streams and rivers, including water quality, water quantity, sediment characteristics, and distribution of water inputs, are subject to alterations that may degrade cave and karst systems. Water quality can be affected by human activities and land use changes (septic systems, non-point-source pollution, and conversion to or from agriculture). The quantity and distribution of waters can be impacted by such things as impervious cover, channelization, sinkhole modification, and storm water diversion. Changing land use patterns around sinkholesand other inputs can radically change the sediment load, balance,
and characteristics of a cave stream. Changes such as these can, in turn, alter the bed characteristics.
Methods
Monitoring of fluvial processes is covered in a separate chapter in this manual. Many of the approaches described in that chapter can also be applied in cave streams. Among the most important parameters for monitoring cave streams are water quantity, turbidity, and water chemical characteristics. Changes in stream bed, in particular siltation or scouring, may also be quite important; however, these may have more effects on biological systems in caves than on the geological resources themselves. In addition, various agencies, including National Park Service Water Resources Division, U.S. Geological Survey Water Resources Program, and the Environmental Protection Agency Office of Water can provide additional guidance and assistance in monitoring cave stream parameters. In addition, references such as U.S. Geological Survey (variously dated) and Krawczyk (1998) provide details on techniques that can be used.
CONCLUSIONS
Developing a monitoring program for a cave, set of caves, or caves and associated karst landscapes is a complex task involving many disciplines. Although this chapter has focused on vital signs that are either geological in nature or which may significantly influence geological processes and resources, a complete monitoring program would also focus on biological resources (including bats, other vertebrates, invertebrates, and microbiology), cultural resources (archaeological and historic), and paleontological resources. In addition, monitoring programs must follow a systematic inventory, which is necessary to identify the resources to be monitored and the potential impacts to them.
The Carlsbad Caverns National Park Cave and Karst Management Plan Environmental Assessment contains a well-developed cave monitoring plan (Carlsbad Caverns National Park, 2006). It discusses the existing monitoring at the park, the rationale used to decide whether new or additional monitoring should take place, and the procedures to be followed in developing new monitoring projects. It provides an excellent guidance for developing cave monitoring projects.
Since monitoring is presumably tied to vulnerable resources, management actions, and needs, it is important to identify the vital signs that are most subject to human impact under existing and potential conditions. The monitoring of some vital signs is likely to be important under a wide variety of conditions. For example, most cave monitoring plans will probably include at least some monitoring of cave microclimate. The reason for this is that cave microclimate may affect a wide range of geological, biological, and cultural resources in a cave. Other types of monitoring may be limited to caves that undergo particular impacts. For example, monitoring of lamp fl ora growth or lint deposition would probably be applicable only in show caves. Water quality monitoring is likely to be more important where a significant threat of contamination exists due to land use or human use of the cave.
Budget is also a significant factor when designing a monitoring program. Fortunately, most of the basic means for monitoring geologic resources in caves do not require expensive, specialized equipment, but they often require either trained or specialized personnel (Table 1). Automating some of the measuring, where possible, may reduce the time requirement for personnel. However, the larger volumes of data from this approach may require personnel with more training to adequately analyze the results.
At few, if any, caves has a monitoring plan been implemented that addresses the entire list of vital signs discussed in this chapter, let alone the biological, microbiological, paleontological, and cultural resource monitoring that would constitute a complete monitoring program. However, this list of vital signs should serve as a guide to what might be monitored, depending on resources needing management, potential and actual threats, budget, and availability of experienced assistance.
ACKNOWLEDGMENTS
This chapter benefited significantly from countless conversations between the author and Rick Olson, Ginger Nolan, Robert Casavant, Douglas Graeme, Johnathan Jernigan, Nick Crawford, Chris Groves, Penny Boston, Warren Campbell, Arrigo Cigna, and a variety of other cave resource managers. Rick Olson, Chuck Bitting, Ron Karpilo, and especially John Roth reviewed the manuscript and thus substantially improved it. Any errors of omission or commission, however, are mine.
REFERENCES CITED
Aley, T., 2004, Tourist caves: algae and lampenflora, in Gunn, J., ed., Encyclopedia of cave and karst science: New York, Fitzroy Dearborn, p. 733–734.
Aley, T., Castillon, K., and Sagendorf, J., 2006, Strategy for managing alpha radiation in show caves to protect caves, cave employees, and cave businesses, in Proceedings, 17th National Cave and Karst Management Symposium, Albany, New York, November 2005: National Cave and Karst Management Symposium Steering Committee, p. 62–71.
Allison, S., 2004, 2004 Lechuguilla Cave Summary: Canyons and Caves, v. 25, p. 2–6.
Baichtel, J.F., and Langendoen, R., 2001, LIDAR applications in a temperate rainforest environment case study: Kosciusko Island southeastern Alaska, Tongass National Forest, in Proceedings, 15th National Cave and Karst Management Symposium, Tucson, Arizona, October 2001: USDA-Forest Service, Coronado National Forest, p. 155.
Baker, A., and Brunsdon, C., 2003, Non-linearities in drip water hydrology: an example from Stump Cross Caverns, Yorkshire: Journal of Hydrology (Amsterdam), v. 277, p. 151–163, doi: 10.1016/S0022-1694(03)00063-5.
Barton, H., and Luiszer, F., 2005, Microbial metabolic structure in a sulfidic cave hot spring: Potential mechanisms of biospeleogenesis: Journal of Caves and Karst Studies, v. 67, p. 28–38.
Barton, H.A., Spear, J.R., and Pace, N.R., 2001, Microbial life in the underworld: Biogenicity in secondary mineral formations: Geomicrobiology Journal, v. 18, p. 359–368, doi: 10.1080/01490450152467840.
Beddows, P.A., Zhang, R., Schwarcz, H.P., and Ford, D.C., 2005, Seasonality of cave drip hydrology and chemistry in relation to mid-latitude North American Holocene paleoclimate record from speleothems: Geological Society of America Abstracts with Programs, v. 37, no. 7, p. 173.
Bhatt, S.K., and Mark, C., 2000, Analysis of safety aspects and mining practices for effective ground control in surface mining, in Peng, S.S., and M.C., eds., Proceedings of the 19th international conference on ground control in mining: Morgantown, West Virginia, West Virginia University, p. 395–404.
Bodenhamer, H., 1996, Monitoring human-caused changes with visitor impact mapping, in Proceedings, 1995 National Cave Management Symposium, Mitchell, Indiana, October 1995: National Cave Management Symposium Steering Committee, p. 28–37.
Bodenhamer, H., 2006, Visitor Impact mapping in caves, in Hildreth-Werker, V. and Werker, J.C., eds., Cave Conservation and Restoration: Huntsville, Alabama, National Speleological Society, p. 193–202.
Brooke, M., 1996, Infiltration pathways at Carlsbad Caverns National Park determined by hydrogeologic and hydrochemical characterization and analysis [master’s thesis]: Golden, Colorado, Colorado School of Mines, 182 p.
Buecher, R.H., 1999, Microclimate study of Kartchner Caverns, Arizona: Journal of Caves and Karst Studies, v. 61, p. 108–120.
Caldwell, D.E., 1991, Limnological survey of cavern pools: Unpublished report to Carlsbad Caverns National Park, 31 p.
Campbell, C.W., and Keith, A.G., 2001, Karst ground-water hydrologic analyses based on aerial thermography: Hydrological Science and Technology, v. 17, p. 59–68.
Campbell, C.W., and Singer, M., 2001, Application of thermography to groundwater monitoring at Arnold Air Force Base, Tennessee: Proceedings—Multidisciplinary Conference on Sinkholes and the Engineering and Environmental Impacts of Karsts, v. 8, p. 352–358.
Campbell, C.W., Abd El Litif, M., and Foster, J.W., 1996, Application of thermography to karst hydrology: Journal of Caves and Karst Studies, v. 58, p. 163–167.
Carlsbad Caverns National Park, 2006, Cave and Karst Management Plan Environmental Assessment, dated 30 September 2006: accessed 10 April 2007 at http://www.nps.gov/cave/parkmgmt/upload/cave_karst_ea_2006.pdf.
Cigna, A.A., 2002, Modern trend in cave monitoring: Acta Cardiologica, v. 31, p. 35–54.
Cigna, A.A., 2004, Climate of caves, in Gunn, J., ed., Encyclopedia of cave and karst science: New York, Fitzroy Dearborn, p. 228–230.
Culver, D.C., 1982, Cave Life: Evolution and Ecology: Cambridge, Massachusetts, Harvard University Press, p. 189.
de Freitas, C.R., and Schmeckal, A., 2005, Prediction of condensation in caves: Speleogenesis and Evolution of Karst Aquifers, v. 3, no. 2, p. 1–9.
Drummond, I., 1992, Photoelectric caver counter: Speleonics, v. 17, p. 8.
DuChene, H.R., 2006, Resource inventory: a tool for cave science, management, and restoration, in Hildreth-Werker, V. and Werker, J.C., eds., Cave conservation and Restoration: Huntsville, Alabama, National Speleological Society, p. 19–32.
Genty, D., and Defl andre, G., 1998, Drip fl ow variations under a stalactite of the Père Noël cave (Belgium): Evidence of seasonal variations and air pressure constraints: Journal of Hydrology (Amsterdam), v. 211, p. 208–232, doi: 10.1016/S0022-1694(98)00235-2.
Gibson, D., 1994, A caver counter: BCRA Cave Radio and Electronics Group Journal, v. 15, p. 24–26.
Gillieson, D., 1996, Caves: processes, development, management: Oxford, UK, Blackwell, p. 324.
Girard, J.M., and McHugh, E., 2000, Detecting problems with mine slope stability: Presentation at 31st Annual Institute on Mining Health, Safety, and Research, Roanoke, Virginia, Aug. 27–30.
Graf, C.G., 1999, Hydrogeology of Kartchner Caverns State Park, Arizona: Journal of Caves and Karst Studies, v. 61, p. 59–67.
Hale, E., 2007, Visitor impact mapping progresses at Oregon Caves: NSS News, v. 65, no. 3, p. 33.
Hamilton-Smith, E., 2002, Management assessment in karst areas: Acta Cardiologica, v. 31, p. 13–20.
Hildreth-Werker, V., 2006, Photographs as cave management tools, in Hildreth-Werker, V. and Werker, J.C., eds., Cave conservation and restoration: Huntsville, Alabama, National Speleological Society, p. 203–216.
Hildreth-Werker, V., and Werker, J.C., eds., 2006, Cave Conservation and Restoration: Huntsville, Alabama, National Speleological Society, p. 600.
Hill, C., and Forti, P., 1997, Cave minerals of the world, second edition: Huntsville, Alabama, National Speleological Society, p. 463.
Howarth, F.G., 1983, Ecology of cave arthropods: Annual Review of Entomology, v. 28, p. 365–389.
Hunter, A.J., Northup, D.E., Dahm, C.N., and Boston, P.J., 2004, Persistent coliform contamination in Lechuguilla cave pools: Journal of Caves and Karst Studies, v. 66, p. 102–110.
Ikner, L.A., Toomey, R.S., III, Nolan, G., Neilson, J.W., Pryor, B.M., and Maier, R.M., 2007, Cultural microbial diversity and the impact of tourism in Kartchner Caverns, Arizona: Microbial Ecology, v. 53, p. 30–42, doi: 10.1007/s00248-006-9135-8.
Imes, J.L., and Fredrick, B.S., 2001, Using dye-tracing and chemical analyses to determine effects of a wastewater discharge to Jam Up Creek on water quality of Big Spring, southeastern Missouri: U.S. Geological Survey Factsheet, FS-103-02, accessed 4 November 2005 at http://mo.water.usgs.gov/ fact_sheets/FS-103-02-Imes/.
Jablonsky, P., Kraemer, S., and Yett, B., 1995, Lint in caves, in Proceedings, National Cave Management Symposium—XI, Carlsbad, New Mexico, October 1993: National Cave Management Symposium Steering Committee, p. 73–81.
James, J.M., 2004, Tourist caves: air quality, in Gunn, J., ed., Encyclopedia of cave and karst science: New York, Fitzroy Dearborn, p. 730–731.
Johnson, S.A., Brack, V., Jr., and Dunlap, R.K., 2002, Management of hibernacula in the state of Indiana, in Kurta, A., and Kennedy, J., eds., The Indiana bat: biology and management of an endangered species: Austin, Texas, Bat Conservation International, p. 100–109.
Jones, W.K., Hobbs, H.H., Wicks, C.M., Currie, R.R., Hose, L.D., Kerbo, R.C., Goodbar, J.R., and Trout, J., eds., 2003, Recommendations and guidelines for managing caves on protected lands: Charles Town, West Virginia, Karst Waters Institute, Special Publication 8.
Krawczyk, W.E., 1998, Manual for karst hydrology: International Journal of Speleology, Handbook 1—Physical Speleology.
Langendoen, R.R., and Baichtel, J.F., 2004, Using LIDAR remote sensing to map karst topography in a temperate rain forest—case study: Tongass National Forest, southeastern Alaska: Geological Society of America Abstracts with Programs, v. 37, no. 7, p. 173.
Luetscher, M., Jeannin, P.-Y., and Haeberli, W., 2005, Ice caves as an indicator of winter climate evolution: a case study from the Jura Mountains: The Holocene, v. 15, p. 982–993, doi: 10.1191/0959683605hl872ra.
McLean, J.S., 1971, The microclimate of Carlsbad Caverns: U.S. Geological Survey Open-File Report 71-198.
McLean, J.S., 1976, Factors altering the microclimate in Carlsbad Caverns: U.S. Geological Survey Open-File Report 76-171.
Michie, N.A., 2001, An instrument and method for measurement of dust fall in caves, in Proceedings, Fourteenth National Cave and Karst Management Symposium, Chattanooga, Tennessee, October 1999: Chattanooga, Southeastern Cave Conservancy, p. 123–128.
Michie, N.A., 2004, Tourist caves: airborne debris, in Gunn, J., ed., Encyclopedia of cave and karst science: New York, Fitzroy Dearborn, p. 731–733.
Montane, J.M., and Whitman, D., 2000, Relationships between microtopography and subsurface karst structures from airborne LIDAR and GPR data: Geological Society of America Abstracts with Programs, v. 32, no. 7, p. 515.
Moore, G.W., and Sullivan, N., 1997, Speleology: caves and the cave environment: St. Louis, Missouri, Cave Books, p. 176.
Musgrove, M., and Banner, J.L., 2004, Controls on the spatial and temporal variability of vadose dripwater geochemistry: Edwards Aquifer, central Texas: Geochimica et Cosmochimica Acta, v. 68, p. 1007–1020.
Myers, D.N., and Wilde, F.D., eds., November 2003, Biological indicators, third edition: U.S. Geological Survey Techniques of Water-Resources Investigations, book 9, chap. A7, accessed 15 November 2005 at http:// pubs.water.usgs.gov/twri9A7/.
Northup, D.E., and Lavoie, K.H., 2001, Geomicrobiology of caves: a review: Geomicrobiology Journal, v. 18, p. 199–222, doi: 10.1080/01490450152467741.
Olson, R.A., 2006, Control of lamp fl ora in developed caves, in Hildreth-Werker, V., and Werker, J.C., eds., Cave conservation and restoration: Huntsville, Alabama, National Speleological Society, p. 343–348.
Onac, B.P., and Vereş, D.Ş., 2003, Sequence of secondary phosphates deposition in a karst environment: evidence from Măgurici Cave (Romania): European Journal of Mineralogy, v. 15, p. 741–745, doi: 10.1127/0935-1221/2003/0015-0741.
Onset Computer Corporation, 2007, Detailed specifi cations for HOBO Pro family of data loggers, accessed 9 April 2006 at http://www.1800loggers. com/solutions/products/loggers/_loggerviewer.php5?pid=478.
Pflitsch, A., and Piasecki, J., 2003, Detection of an airfl ow system in Niedzwiedzia
(Bear) Cave, Kletno, Poland: Journal of Caves and Karst Studies, v. 65, p. 160–173.
Powell, G.M., and Rogers D., 2005, Measuring the depth to water in wells: Kansas State University Agricultural Experiment Station and Cooperative Extension Service, MF 2669.
Sanz, E., and Lopez, J.J., 2000, Infi ltration measured by the drip of stalactites: Ground Water, v. 38, p. 247–253, doi: 10.1111/j.1745-6584.2000.tb00336.x.
Sasowsky, I.D., and Dalton, C.T., 2005, Measurement of pH for fi eld studies in karst areas: Journal of Caves and Karst Studies, v. 67, p. 127–132.
Seale, L.D., Brinkmann, R., and Vacher, H.L., 2004, Creating a sinkhole GIS database: can ALSM detect sinkholes in urbanized Florida: Geological Society of America Abstracts with Programs, v. 36, no. 5, p. 299.
Thompson, J., and Marvin, M., 2006, Experimental Research on the Use of Thermography to Locate Heat Signatures from Caves, in Proceedings, 17th National Cave and Karst Management Symposium, Albany, New York, November 2005: National Cave and Karst Management Symposium Steering Committee, p. 102–115.
Thornhill, J.T., 1989, Ground-water issue paper: accuracy of depth to water measurements: U.S. Environmental Protection Agency, EPA/540/4-89/002, 3 p.
Toomey, R.S., III, 2005, Kartchner Caverns State Park Environmental and Research Report 2005: Report Submitted to the Arizona State Parks Board, 15 September 2005, p 1–25.
Toomey, R.S., III, and Nolan, G., 2005, Environmental change at Kartchner Caverns: trying to separate natural and anthropogenic changes, in Conference Proceedings of Biodiversity and Management of the Madrean Archipelago II: Connecting Mountain Islands and Desert Seas, USDA Forest Service Proceedings RMRS-P-36, p. 264–270.
Toomey, R.S., III, Taylor, S.J., Tecic, D., Newman, D., and Hespen, C., 2001, The potential use of data-logging light intensity and light on/off meters for mapping visitor use of wild caves, in Proceedings, Fourteenth National Cave and Karst Management Symposium, Chattanooga, October 1999: Chattanooga, Southeastern Cave Conservancy, p. 199.
U.S. Geological Survey, variously dated, National fi eld manual for the collection of water-quality data: U.S. Geological Survey Techniques of Water-Resources Investigations, book 9, chapters A1–A9, available online at http://water.usgs.gov/owq/FieldManual/ (accessed 10 February 2009).
van der Heijde, P.K.M., Kolm, K.B., Dawson, H., and Brooke, M., 1997, Determining water infiltration routes from buildings located above Carlsbad Cavern, Carlsbad Caverns National Park, Carlsbad, New Mexico: Golden, Colorado, International Groundwater Modeling Center report IGWMCGWMJ 97-01, 88 p.
Veni, G., DuChene, H., Crawford, N.C., Groves, C.G., Huppert, G.H., Kastning, E.H., Olson, R., and Wheeler, B.J., 2001, Living with karst: a fragile foundation: Environmental awareness series: Alexandria, Virginia, American Geological Institute, p. 64.
Werker, J., and Hildreth-Werker, V., 1996, New improved system of photomonitoring, in Proceedings, 1995 National Cave Management Symposium, Mitchell, Indiana, October 1995: National Cave Management Symposium Steering Committee, p. 311–312.
White, E.L., 2005, Breakdown, in Culver, D.C., and White, W.B. eds., Encyclopedia of caves: Boston, Elsevier Academic Press, p. 56–60.
White, W.B., 2005, Springs, in Culver, D.C., and White, W.B. eds., Encyclopedia of caves: Boston, Elsevier Academic Press, p. 565–570.
Yonge, C.J., 2004, Ice in caves, in Gunn, J., ed., Encyclopedia of cave and karst science: New York, Fitzroy Dearborn, p. 435–437.