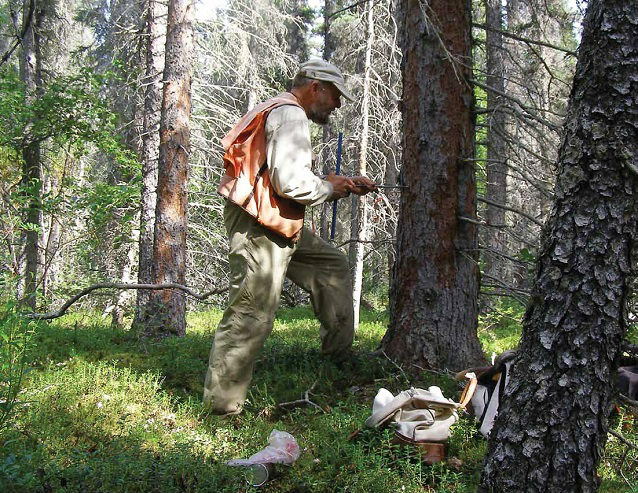
NPS photograph
Trees represent one of the most important records of environmental change on Earth. Tree growth, as recorded in the width of annual rings, can be useful for identifying the local to global effects of volcanic eruptions, and for comparing these effects with historical records across large areas. Globally, a major eruption can lead to short-term changes in climate that can have measurable effects on tree growth. Volcanic dust and sulfur-based aerosols released into the stratosphere can absorb incoming solar radiation, resulting in cooler than average summer temperatures and warmer than average winters for one or more years following an eruption. Closer to the eruption site, strong winds, crown scorch, and heavy ash fall can cause physical injury to trees and inhibit photosynthesis for months.
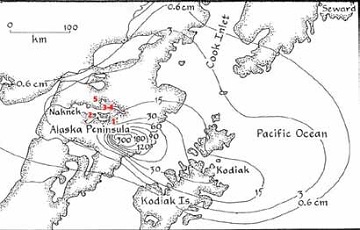
When environmental conditions change abruptly, trees typically show either reduced growth (‘growth suppression’) or accelerated growth (a ‘growth release’) that is measured by changes in the width of annual rings. Tree growth rates generally decline with age, but a growth suppression is indicated by a rapid (e.g., 1-3 year) decrease in growth rate, generally in response to an unfavorable climate, or to a disturbance that temporarily inhibits growth but does not kill the tree. In contrast, growth releases can be detected in surviving trees when neighboring trees die and release the remaining trees from competition for light and nutrients. For example, a growth release could occur in understory trees if larger, over story trees were knocked down by wind or killed by insects or another type of disturbance. A growth release could also occur if conditions became more favorable for growth, e.g., due to an increase in growing-season temperature or nutrient availability.
The eruption of Novarupta on June 6-8, 1912, produced an estimated four cubic miles (17 km3) of ash fallout (Fierstein and Hildreth 1992) and resulted in one of the most significant cooling events of the twentieth century (Briffa et al. 1998). Colder than average temperatures persisted for more than six months after the eruption (Mass and Portman 1989) and were reflected in reduced growth and/or frost damage (‘frost rings’) in tree-rings across the Northern Hemisphere (Briffa et al. 1998, LaMarche and Hirschboeck 1984).
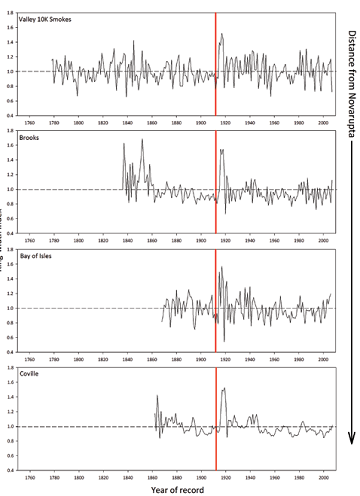
Tree-ring data from white spruce (Picea glauca) stands in Katmai (Figure 1) indicate that the effects of the Novarupta eruption were two-fold. The data were collected by biologists from NPS, Humboldt State University, and the U.S. Fish and Wildlife Service as part of a larger study of forest disturbance (Sherriff et al. 2011). Immediately following the eruption, trees experienced an abrupt, short-term decrease in growth, consistent with records that suggest widespread cooling (Briffa et al. 1998). However, the growth suppression appeared only in the stands sampled in Katmai and not in other locations sampled (e.g., farther north on the Alaska Peninsula or east on the Kenai Peninsula). This suggests that localized effects, such as damage due to ash fall and/or continued seismic activity, played a greater role than climate in slowing tree growth.
A dramatic shift occurred after the Novarupta eruption, when a short-lived (less than 10 years) but significant period of rapid growth began (Figure 2) (Eicher and Rounsefell 1957). Between 20% and 80% of trees sampled in Katmai showed an increase in growth during this time, as did trees downwind from the eruption site, on Kodiak Island (Kaiser and Kaiser-Bernhard 1987). Similar growth releases have also been reported one to several years following the eruption of Volcán Llaima, Chile, in 1640 (Pollman 2003);Volcán de Fuego de Colima, Mexico, in 1913 (Biondi et al. 2003); and Mount St. Helens, Washington, in 1980 (Yamaguchi and Lawrence 1993, Segura et al. 1995, Weber et al. 2006). This ‘rebound effect’ indicated by an increase in growth is usually ascribed to low-level disturbance (e.g., tephra deposits or lahar flows) that opens the canopy.
The localized growth release that followed the 1912 eruption of Novarupta occurred shortly after the start of a regional-scale release that has been attributed to spruce beetle disturbance. The Katmai records, described above, were compiled by the NPS and cooperators as part of a larger, regional tree-ring dataset that spans a roughly 250-year period (Sherriff et al. 2011). Large-scale spruce beetle outbreaks were recorded in the 1810s, 1870s, and 1970s, as well as in the early 1900s, shortly before the 1912 eruption (Figure 3). The short-lived and highly synchronous growth release recorded in the Katmai tree cores after 1912 (Figure 2) was not observed at other sites in the study and was therefore excluded from the regional spruce beetle analysis. The short-lived suppression and subsequent growth release were, however, recorded in tree-ring records from Kodiak, compiled as part of a separate study (Kaiser and Kaiser-Bernhard 1987). Ash depths in Kodiak were as great as 12 inches (30 cm), whereas upwind, near Lake Brooks and several of our study sites, they ranged from 4-8 inches (10-20 cm) (Fierstein and Hildreth 1992).
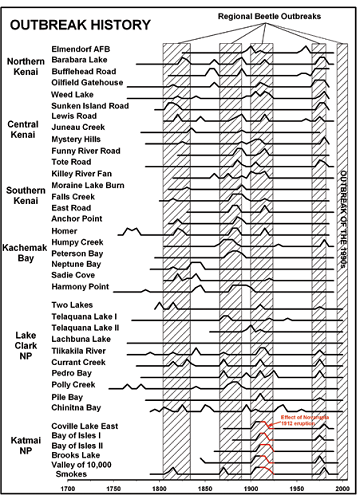
The cause of the post-1912 release in Katmai and Kodiak is uncertain, but there are several possible explanations. Some authors have suggested that it could have resulted from a fertilization effect associated with ash fall (Eicher and Rounsefell 1957). This is unlikely a direct effect of ash deposition, as the silica-rich ash was extremely nutrient-poor (Griggs 1920, Williams and McBirney 1979), comparable in nutrient content to finely-ground glass. Instead, any increase in soil nutrient availability would have been due to soil disturbance that stimulated microbial activity, an increase in soil organic matter due to the decay of buried plants, and/or reduced competition for nutrients due to the decline and/or death of some trees.
Similarly, canopy gaps resulting from crown damage or wind throw could have increased light availability to understory and/or neighboring trees, releasing them from competition and enhancing their growth. It is also possible that moderate ash fall could provide a mulching effect (cf. Segura et al. 1995), enhancing soil moisture, similar to plastic sheeting on a garden. The fact that the stands in Katmai were already under attack by spruce beetles at the time of the eruption suggests that the trees were drought-stressed. Thus, an increase in soil moisture retention could have facilitated tree growth.
Trees at most of the sites in Katmai range in age from 130-170 years, having established between 1860 and 1890. However, the study site closest to the eruption site, located along the Valley of 10,000 Smokes Road, also has trees that established between 1900 and 1920, suggesting that ash fall did not adversely affect seedlings and may have in fact facilitated establishment. Living trees as old as 235 years were also found at the site, indicating an available seed source at the time of the eruption.
The interaction of spruce beetle disturbance and volcanic activity has likely affected forest structure throughout southern Katmai, with volcanism affecting the growth and expansion of spruce forests on Kodiak, as well. Tree-ring chronologies, stand age and structure, and climate data can be used in concert to understand long-term forest dynamics and to make predictions about future forest conditions. As additional tree-ring records from the region are analyzed, biologists will continue to reconstruct the influence of past eruptions on tree growth and climate, and to analyze forest responses to environmental change, both of which can provide a basis for understanding future responses to rapid changes in climate.
References
Biondi, F., I.G. Estrada, J.C.G. Ruiz, and A.E. Torres. 2003. Tree growth response to the 1913 eruption of Volcán de Fuego de Colima, Mexico. Quaternary Research 59: 293-299.
Briffa, K.R., F.H. Schweingruber, P.D. Jones, T.J. Osborn, S.G. Shiyatov, and E.A. Vaganov. 1998. Reduced sensitivity of recent tree-growth to temperature at high northern latitudes. Nature 391: 678-682.
Eicher, G.J., Jr., and G.A. Rounsefell. 1957. Effects of lake fertilization by volcanic activity on abundance of salmon. Limnology and Oceanography 2: 70-76.
Fierstein, J., and W. Hildreth. 1992. The plinian eruptions of 1912 at Novarupta, Katmai National Park, Alaska. Bulletin of Volcanology 54: 646-684.
Griggs, R.F. 1920. The recovery of vegetation at Kodiak. Ohio State University Bulletin 24: 1-57.
Kaiser, K.F., and C. Kaiser-Bernhard. 1987. The Katmai eruption of 1912 and the Alaska earthquake of 1964 as reflected in the annual rings of Sitka Spruce on Kodiak Island. Dendrochronologia 5: 111-125.
LaMarche, V.C., Jr., and K.K. Hirschboeck. 1984. Frost rings in trees as records of major volcanic eruptions. Nature 307: 121-126.
Mass, C.F., and D.A. Portman. 1989. Major volcanic eruptions and climate: A critical evaluation. Journal of Climate 2: 566-593.
Pollman, W. 2003. Stand structure and dendroecology of an old-growth Nothofagus forest in Conguillio National Park, south Chile. Forest Ecology and Management 176: 87-103.
Segura, G., T.M. Hinckley, and L.B. Brubaker. 1995. Variations in radial growth of declining old-growth stands of Abiesamabilis after tephra deposition from Mount St. Helens. Canadian Journal of Forest Research 25: 1484-1492.
Sherriff, R.L., E.E. Berg, and A.E. Miller. 2011. Climate variability and spruce beetle (Dendroctonus rufipennis) outbreaks in southcentral and southwest Alaska. Ecology 92: 1459-1470.
Weber, M.H., K.S. Hadley, P.M. Frenzen, and J.F. Franklin. 2006. Forest development following mudflow deposition, Mount St. Helens, Washington. Canadian Journal of Forest Research 36: 437-449.
Williams, H., and A.R. McBirney. 1979. Volcanology. Freeman, Cooper and Company. San Francisco.
Yamaguchi, D.K., and D.B. Lawrence. 1993. Tree-ring evidence for 1842-1843 eruptive activity at the Goat Rocks dome, Mount St. Helens, Washington. Bulletin of Volcanology 55: 264-272.
Part of a series of articles titled Alaska Park Science - Volume 11 Issue 1: Volcanoes of Katmai and the Alaska Peninsula.
Last updated: July 7, 2016