Part of a series of articles titled Park Paleontology News - Vol. 15, No. 1, Spring 2023.
Article
Utilizing Trace Fossils to Fill in Paleoecological Gaps at Curecanti National Recreation Area
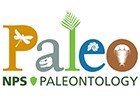
Ryan King, PhD
Natural & Environmental Sciences Department, Western Colorado University

NPS photo by Victoria Stauffenberg.
Introduction
Curecanti National Recreation Area (CURE) occupies a forty-mile stretch along the Gunnison River between Montrose and Gunnison, Colorado (Figure 1) with outcrops of the Morrison Formation, one of the most prolific dinosaur-bearing units in the United States (Tweet et al., 2012). While outcrops of the Upper Jurassic Morrison Formation are often hard to access in CURE, some body fossils (bones and teeth) from sauropods (Camarasaurus, Apatosaurus, and other diplodocids), theropods (Ceratosaurus, Allosaurus), stegosaurs, turtles, crocodilians, and probable mammal material have been recovered from the area in the last thirty years (Fiorillo and May, 1996; Fiorillo et al., 1996; Trujillo, 2000; Koch et al., 2006). Additionally, trace fossils such as the burrows of invertebrates (termites, bees, crawfish, earthworms, and bivalves) have been observed (Fiorillo and Hasiotis, 1996; Fiorillo, 1999; Fiorillo and Harris, 2000). Body fossils are regarded as a fossilized part of the organism, whereas trace fossils are not part of the organism, but a fossilized biogenic trace that shows the organism was present.

The Importance of Trace Fossils
When you see a mounted dinosaur skeleton while entering a museum, it is easy to imagine how body fossils tell us about the past, but there is a much larger ancient ecosystem that was present than for which body fossils account. In lieu of body fossils, trace fossils help us fill in that paleoecological gap, and help us understand how organisms interacted with their environment, and sometimes with each other. Trace fossils are useful in this endeavor because many organisms have one single set of hard parts (e.g., body fossils: bones, shells, etc.), but an organism may create many trace fossils in its lifetime. For instance, you don’t need a dinosaur to pass away in a location to see that it walked through or lived in that environment. Additionally, in some cases, the taphonomy (postmortem processes) of some environments do not lend to the preservation of bones or hard parts. Even more so, trace fossils are helpful because they are dominantly found in situ (or in place), whereas wind or more likely water may readily move body fossils (e.g., leaves, bones, shells). The common disassociation of body fossils from trace fossils produced by the organism creates a bit of a disconnect in that scientists then have to figure out what organism made the trace fossil. Furthermore, many organisms may create the same structures (trace fossils) over time as a convergence of behavior (Ekdale et al., 1984). Behaviors are convergent because organism often exploit environmental conditions for protection and food in similar manners (niche filling). Alternatively, since the Jurassic some trace fossils are similar because the organism’s lineages (e.g., turtle, crocodiles, insects) are long ranging.
What Trace Fossils in Curecanti National Recreation Area Tell Us
Trace fossils are often representative of organism behavior, and in this sense we can use trace fossils to inform us about environmental conditions, as well as what organism may have made the trace fossil, by utilizing what we know from modern biology about organism behavior (uniformitarianism). Much like body fossils, trace fossils are given names that indicate a repeatable structure. For instance, the small meniscate structures seen in CURE are often referable to the trace fossil names Beaconites or Taenidium (Bradshaw, 1981; Smith et al., 2008) (Figure 2A).

Figure 2: Examples of relating modern tracemakers to fossilized traces observed in the Morrison Formation of Curecanti National Recreation Area : A) modern burrowing bugs and cicadas often feed on roots, excavating in front and backfilling with sediment in meniscate packages (top, after examples of Smith et al., 2008) of which these meniscate structures can often be seen as trace fossils such as Beaconites or Taenidium (two examples, bottom), B) conversely, mayflies live in the water for much of their life and utilize U-shaped burrows (top) to pump water (blue arrows) with food in one end and waste out the other end; movement of the open U-shaped structure over time results in a time-averaged trace fossil such as Fuersichnus (bottom, packages of adjacent U-shapes), C) similarly, unionid bivalves live in the water and filter feed, but draw the water in/waste out of their body (top, blue arrows); the muscular foot and sometimes the outline of the shell create almond-shaped traces in the sediment; these bivalves often align with the current (potentially due to less drag or feeding/waste disposal reasons) which results in the fossilized traces (Lockeia) appearing in similar alignment when viewing the upper or lower surface of beds (bottom). Scale bars in photos are 3 cm (1.2 inches) long.
The tracemakers of these burrows in the modern are typically burrower bugs (Cydnidae) and cicadas which often feed on roots (Hasiotis, 2004; Smith et al., 2008). This suggest insects and roots were present and that this sediment was subaerially exposed. Conversely, the U-shapes that look banana-like in appearance (Figure 2B) are that of the trace fossil Fuersichnus (Bromley and Asgaard, 1979). Fuersichnus is believed to be produced by mayflies (Hasiotis, 2004; Bolotov et al., 2022). This suggests the sediment was subaqueous at some point because mayflies spend most of their lives in water and use these U-shaped tubes for protection and feeding purposes (Scott et al, 1959; Uchman et al., 2016; Savrda, 2019). Over time the organism moves the structure creating a composite, time-average structure of this movement, which looks more banana-like in overall appearance. Another trace fossil seen in CURE on the tops or bases of sandstone are Lockeia (James, 1879; Maples, 1989) (Figure 2C), which has an almond shape in plan view. This represents the bottom of a bivalve (typically the structure created by the pressing down of the muscular foot). This trace fossil tells us that the environment was subaqueous, and suggests the water was moving (river) because the traces are oriented in a similar direction. This is seen in modern bivalves to reduce the drag on the shell and so that upstream the food goes into the inhalant siphon and downstream waste comes out the exhalent siphon (Fiorillo, 1999; Nara, 2003). Where possible, as paleontologists we try to reconstruct the paleoenvironments utilizing inferences such as these gained from the collection of multiple traces fossils within a sedimentary bed. These assemblages of trace fossils are often repeatable because the environmental conditions are repeatable through time and linked to specific environments (e.g., river, floodplain, bay, delta, beach)(Seilacher, 1967; Hasiotis, 2004; MacEachern et al., 2007).
Trace fossils can help inform us on the paleoecology of organism that have soft parts with limited preservation potential, but what information does this provide when we already have the body fossil of the organism? By comparing the foot structure of the bones to tracks that are seen in CURE containing three digit markings (Figure 3), it is possible to see both paleoecological implications, as well as information about the animal itself.

Figure 3: Outline of vertebrate foot bones (body fossils, left) compared with tracks (trace fossils) that show the preservation of three digits represented in photographs (middle) and black and white Digital Elevation Models (right): A) bones of theropod Compsognathus (modified from Thulborn, 1990; Kurzanov, 1987) compared with theropod track shows that much of the foot did not impress because many Upper Jurassic theropods walked in a digitigrade posture, B) conversely Stegosaurus (modified from Thulborn, 1990; Gilmore, 1914) has foot bones that are much shorter than the associated fossilized track examples, which suggest that stegosaurs had a large fleshy pad in the posterior part of the foot, C) sometimes the footprint is more representative of a behavior rather than a more static imprint, as seen with the crocodilian foot of Goniopholis (modified from Erickson, 2016) and the crocodilian trace fossil representing partially buoyant swimming of the animal. Scale bars in photos are 10 cm (3.9 inches) long.
The Grallator-like (Lockley, 2009) tracks of a small theropod (Figure 3A) shows only the impressions of the distal portion of the foot. While preservation of tracks is often reliant on the nature of the substrate, this trait is repeatable in theropod tracks throughout the Morrison Formation and is indicative of a digitigrade posture, which means the animal walked with much of its weight on its toes (Wilson et al., 2009). Conversely, the stegosaur tracks (Deltapodus, Whyte and Romano, 1994; 2001) in CURE have an elongate heel as compared to the foot bones (Figure 3B), which has been postulated in large animals to represent a large posterior pad in the foot for weight support (e.g., supportive heel tissue in sauropod feet: Thulborn, 1990; Jannel et al., 2022). Alternatively, the Hatcherichnus (Foster and Lockley, 1997; Avanzini et al., 2010) tracks (Figure 3C) in CURE do not mimic the shape of the outline of the foot, but they represent the structure left behind by a partially buoyant crocodilian swimming with the tips of digits contacting the substrate as it moved along (Foster and Lockley, 1997).
Aside from providing information on the overall shape or behavior related to parts of the animal, sometimes extraordinary detail of the soft parts of the animal are recorded in passing. Rare examples of skin impression are observed in CURE, often associated with tracks (Figure 4).

Skin impression are larger 5-6 sided polygons (Figure 4A,B) or small rounded features (Figure 4C). The preservation of the skin is important to understand, but the skin itself may tell you about which animal produced it, as the larger polygons are often associated with sauropods (Lockley et al., 2008).
Conclusion
Curecanti National Recreation Area contains a wealth of invertebrate and vertebrate trace fossils that will help to build paleoecological representations of the Jurassic landscape. Continued surveying of Morrison deposits and documentation in CURE will build upon the results presented herein that are just beginning to scratch the surface of the valuable paleontological resources within this area.
Acknowledgements
Thanks to the Curecanti National Recreation Area National Park Service Staff (including Forest Frost and Alison Mims), Spenser Mehew, John Foster, Tara Allman, and Matt King for their contributions.
References
-
Avanzini, M., Piñuela, L., Ruiz-Omeñaca, J.I. and Garcia-Ramos, J.C. 2010. The crocodile track Hatcherichnus, from the Upper Jurassic of Asturias (Spain). in Milàn, J, Lucas, S.G., Lockley, M.G., and J.A., editors. Crocodyle tracks and traces. New Mexico Museum of Natural History and Science, Albuquerque, NM. Bulletin 51.
-
Bolotov, I.N., Kondakov, A.V., Potapov, G.S., Palatov, D.M., Chan, N., Lunn, Z., Bovykina, G.V., Chapurina, Y.E., Kolosova, Y.S., Spitsyna, E.A. and Spitsyn, V.M. 2022. Bioerosion of siliceous rocks driven by rock-boring freshwater insects. npj Materials Degradation, 6(3), 1-23.
-
Bradshaw, M.A. 1981. Paleoenvironmental interpretations and systematics of Devonian trace fossils from the Taylor Group (Lower Beacon Supergroup), Antarctica. New Zealand Journal of Geology and Geophysics, 24: 615–652.
-
Bromley, R. and Asgaard, U. 1979. Triassic freshwater ichnocoenoses from Carlsberg Fjord, east Greenland. Palaeogeography, Palaeoclimatology, Palaeoecology, 28: 39-80.
-
Ekdale, A.A., Bromley, R.G. and Pemberton, S.G. 1984: Ichnology, Trace Fossils in Sedimentology and Stratigraphy: Society for Sedimentary Geology Short Course Notes 15, 316 p. SEPM, Tulsa.
-
Erickson, B.R., 2011. A new skeleton of the neosuchian crocodyliform Goniopholis with new material from the Morrison Formation of Wyoming. Science Museum of Minnesota, Monograph in Paleontology, 10: 29 p.
-
Fiorillo, A.R. 1996. Paleontologic resources in the Upper Jurassic Morrison Formation of Curecanti National Recreation Area and Black Canyon of the Gunnison National Monument, Colorado. Final Report, 105-116.
-
Fiorillo, A.R. 1999. Non-marine trace fossils from the Morrison Formation (Jurassic) of Curecanti National Recreation Area, Colorado. Pages 42-46 in Santucci, V.L. and L. McClelland, editors. NPS Paleontological Research Volume 4. Technical Report NPS/NRGRD/GRDTR-99/03.
-
Fiorillo, A.R. and Harris, R.L. 2000. Late Jurassic (Morrison Formation) continental trace fossils from Curecanti National Recreation Area, Colorado. Park Science 20(2):28-29, 35.
-
Fiorillo, A.R. and Hasiotis, S.T. 1996. Preliminary report on the continental ichnofossils from the Upper Jurassic Morrison Formation of Curecanti National Recreation area, Colorado. Abstracts with Programs - Geological Society of America 28(4): 7-8.
-
Fiorillo, A.R. and May, C.L. 1996. Preliminary report on the taphonomy and depositional setting of a new dinosaur locality in the Morrison Formation (Brushy Basin Member) of Curecanti National Recreation Area, Colorado. Pages 555-561 in Morales, M., editor. The continental Jurassic. Museum of Northern Arizona, Flagstaff, AZ. Bulletin 60.
-
Foster, J.R., and Lockley, M.G., 1997. Probable crocodilian tracks and traces from the Morrison Formation (Upper Jurassic) of eastern Utah. Ichnos, 5:121-129.
-
Gilmore, C.W. 1914. Osteology of the armored Dinosauria in the United States National Museum, with special reference to the genus Stegosaurus. United States National Museum Bulletin 89: 1–136.
-
Hasiotis, S.T. 2004. Reconnaissance of Upper Jurassic Morrison Formation ichnofossils, Rocky Mountain Region, USA: paleoenvironmental, stratigraphic, and paleoclimatic significance of terrestrial and freshwater ichnocoenoses. Sedimentary Geology 167(3-4):177-268.
-
James, U.P., 1879, Description of new species of fossils and remarks on some others, from the Lower and Upper Silurian rocks of Ohio. The Paleontologist, 3: 17-24.
-
Jannel, A., Salisbury, S.W. and Panagiotopoulou, O., 2022. Softening the steps to gigantism in sauropod dinosaurs through the evolution of a pedal pad. Science Advances, 8(31): p.eabm8280.
-
Koch, A. L., Frost, F. and K.C. Trujillo. 2006. Paleontological discoveries at Curecanti National Recreation Area and Black Canyon of the Gunnison National Park, Upper Jurassic Morrison Formation, Colorado. Pages 35-38 in Foster, J. R. and S. G. Lucas, editors. Paleontology and geology of the Upper Jurassic Morrison Formation. New Mexico Museum of Natural History and Science, Albuquerque, NM. Bulletin 36.
-
Kurzanov, S.M. [Avimimids and the problem of the origin of birds.] Sovmestnaia Sovestsko-Mongol'skaia Paleontologicheskaia Ekspeditsiia Trudy, 31: 1-92.
-
Lockley M., Garcia-Ramos J.C., Pinuela L., Avanzini M. 2008. A review of vertebrate track assemblages from the Late Jurassic of Asturias, Spain with comparative notes on coeval ichnofaunas from the western USA: implications for faunal diversity in siliciclastic facies assemblages. Oryctos, 8: 53–70
-
Lockley, M.G. 2009. New perspectives on morphological variation in tridactyl footprints: clues to widespread convergence in developmental dynamics. Geological Quarterly, 53(4): 415-432.
-
MacEachern, J.A., Bann, K.L., Pemberton, S.G., and Gingras, M.K., 2007. The ichnofacies paradigm: high-resolution paleoenvironmental interpretation of the rock record. in MacEachern, J.A., Bann, K.L., Gingras, M.K., Pemberton, S.G., editors, Applied Ichnology. SEPM Short Course Notes, 52: 27-64.
-
Maples, C.G. and West, R.R. 1989. Lockeia, not Pelecypodichnus. Journal of Paleontology, 63(5): 694-696.
-
Nara, M. 2003. Preferentially oriented Lockeia siliquaria in the Miocene Tatsukushi Formation, southwestern Japan : Paleoecology and sedimentological significance of infaunal suspension-feeding bivalves. Journal-Geological Society of Japan. 109: 710–721.
-
Savrda, C.E. 2019. Bioerosion of a modern bedrock stream bed by insect larvae (Conecuh River, Alabama): implications for ichnotaxonomy, continental ichnofacies, and biogeomorphology. Palaeogeography, Palaeoclimatology, Palaeoecology, 513: 3–13.
-
Scott, D.C., Berner, L., and Hirsch, A. 1959. The nymph of the mayfly Genus Tortopus (Ephemeroptera: Polymitarcidae). Annals of the Entomological Society of America, 52: 205–213.
-
Seilacher, A. 1967. Bathymetry of trace fossils. Marine Geology, 5: 413–428.
-
Smith, J.J., Hasiotis, S.T., Kraus, M.J. and Woody, D.T. 2008. Naktodemasis bowni: new ichnogenus and ichnospecies for adhesive meniscate burrows (AMB), and paleoenvironmental implications, Paleogene Willwood Formation, Bighorn Basin, Wyoming. Journal of Paleontology, 82(2):.267-278.
-
Thulborn, R.A. 1990. Dinosaur tracks. Chapman and Hall, London. 410 p.
-
Trujillo, K. 2000. Dino Cove Paleontological Investigations, 1994-1999, Curecanti National Recreation Area: Curecanti National Recreation Area Report, 1-21.
-
Tweet, J. S., V. L. Santucci, T. Connors, and J. P. Kenworthy. 2012. Paleontological resource inventory and monitoring: Northern Colorado Plateau Network. Natural Resource Technical Report NPS/NCPN/NRTR—2012/585. National Park Service, Fort Collins, Colorado.
-
Uchman, A., Mikuláš, R., and Stachacz, M., 2017, Mayfly burrows in firmground of recent rivers from the Czech Republic and Poland, with some comments on Ephemeropteran burrows in general. Ichnos, 24: 191–203.
-
Whyte, M and Romano, M. 1994. Probable sauropod footprints from the Middle Jurassic of Yorkshire, England. Gaia, 10:15–26.
-
Whyte, M.A. and Romano, M., 2001. Probable stegosaurian dinosaur tracks from the Saltwick Formation (Middle Jurassic) of Yorkshire, England. Proceedings of the Geologists' Association, 112(1): 45-54.
-
Wilson J.A., Marsicano C.A., Smith R.M.H. 2009. Dynamic Locomotor Capabilities Revealed by Early Dinosaur Trackmakers from Southern Africa. PLoS ONE 4(10): e7331.
Related Links
-
Curecanti National Recreation Area, Colorado—[Geodiversity Atlas] [Park Home] [npshistory.com]
Last updated: March 22, 2023