Part of a series of articles titled Reckoning with a Warming Climate.
Article
How Wildlife are Responding to a Warming Climate
RESEARCH REVIEW
Nina Chambers, Science Communicator, Alaska Region and Inventory & Monitoring Division, National Park ServiceMaggie MacCluskie, Program Manager, Central Alaska Network, National Park Service
Krista Bartz, Aquatic Ecologist, Southwest Alaska Network, National Park Service
Bridget Borg, Wildlife Biologist, Denali National Park and Preserve, National Park Service
Matthew Cameron, Wildlife Biologist, Gates of the Arctic National Park and Preserve/ Yukon-Charley Rivers National Preserve, National Park Service
William Deacy, Wildlife Biologist, Arctic Network, National Park Service
Grant Hilderbrand, Associate Regional Director for Resources, Alaska Region, National Park Service
Kyle Joly, Wildlife Biologist, Gates of the Arctic National Park and Preserve/Yukon-Charley Rivers National Preserve, Arctic Network, National Park Service
Amy Larsen, Aquatic Ecologist, Gates of the Arctic National Park and Preserve/Yukon-Charley Rivers National Preserve, Arctic Network/Central Alaska Network, National Park Service
Tania Lewis, Wildlife Biologist, Glacier Bay National Park and Preserve, National Park Service
Buck Mangipane, Natural Resource Program Manager, Lake Clark National Park and Preserve, National Park Service
Carol McIntyre, Wildlife Biologist, Denali National Park and Preserve, National Park Service
Jeremy Mizel, Wildlife Biologist, Arctic Network and Central Alaska Network, National Park Service
Pat Owen, Wildlife Biologist, Denali National Park and Preserve, National Park Service
David Payer, Regional Wildlife Biologist, Alaska Region, National Park Service
Joshua Schmidt, Biometrician, Central Alaska Network, National Park Service
Mathew Sorum, Wildlife Biologist, Gates of the Arctic National Park and Preserve/Yukon-Charley Rivers National Preserve, Arctic Network and Central Alaska Network, National Park Service

NPS/TANIA LEWIS
Alaska spans wide latitudinal and elevational ranges, leading to a diverse array of habitat types from temperate coastal rain forests to alpine and Arctic tundra. Therefore, the corresponding impacts of climate change and their implications to wildlife also vary greatly. In addition to warming temperatures and changes in precipitation, climate-driven extremes and events like heavy snowfall, rain-on-snow, and even drought have affected many parks in recent years. Below we describe our observations from research and monitoring studies conducted in Alaska parks and provide context for those observations where possible.
Some species adapt to environmental conditions quickly.
- Insects and wood frogs are species that respond quickly to environmental conditions and cues. Some shorebird species and caribou have adapted their timing for migration to take advantage of changing conditions, while other species like wolves are having pups earlier.
- Some shorebirds have adapted to earlier spring insect emergence. Shorebirds migrate to Alaska in the spring to nest and raise young when they can take advantage of a plentiful food source: insects. Insect populations respond quickly to environmental conditions; they emerge based on snow melt and warming air temperatures. Across Arctic North America, insects are emerging an average of 1-2.5 days earlier per decade. In response, some shorebird species have adapted to match this timing by arriving to their breeding grounds earlier. Other shorebird species have adapted by adjusting their egg-laying to be within a shorter time period after they arrive (Shaftel et al. 2021). In some cases, populations of insects that shorebird adults and chicks eat peaked before the chicks hatched, resulting in a “trophic mismatch” through reduced food availability when energy demands are greatest for offspring. The degree of mismatch between insect availability and demand for insects by nestlings varied by location and species (Kwon et al. 2019).
- Frogs are nimble in adapting to environmental conditions. Wood frogs have one of the most widespread ranges of frogs in North America—from the southeastern U.S. to the Canadian subarctic and as far north as the Brooks Range in Arctic Alaska. It is the only amphibian found this far north and has a surprising adaptation to the cold—it freezes in the winter and thaws out to carry on with life in the spring. Like many species of wildlife, amphibians time their breeding season to correspond to optimal conditions for success. Warmer air temperatures and less snow cover have changed the amount of spring runoff and reduced the amount of aquatic breeding habitat. Using acoustic monitoring from 2011-2017, we found a strong relationship between frog-calling activity and temperature and snowpack. Monitoring is ongoing, but our findings so far suggest that wood frogs will rapidly adjust the timing of their breeding (Larsen et al. 2021).

NPS/REMOTE CAMERA
- Some wolves may be denning earlier; if they don’t, they could be missing out. Wolves use den sites for giving birth and to provide a protected area for young pups and nursing females. A study that looked at wolf denning patterns over 25 years determined that wolves in east-central Alaska may be denning earlier in the spring in response to warmer weather and food availability for the new pups (Joly et al. 2018). Another study that looked at eight populations of wolves across North America between 2000 and 2017 found that the onset of spring shifted a full two weeks earlier, but the average denning date did not change, which highlights the complexity inherent with localized adaptations to changing climate conditions (Mahoney et al. 2020).
Some species benefit in the short term from warmer temperatures and earlier growing seasons.
Some species benefit from warmer weather and an earlier, longer growing season. What we don’t know yet is how warm it can get and still be beneficial.
- Insects thrive in a warming climate and increased insect harassment stresses caribou. As the climate warms, some insects thrive. When caribou are harassed by insects, they tend to move more and eat less (Joly et al. 2020, Ehlers et al. 2021). Warm, windless summers that favor insects lead to poorer maternal health and delayed arrival at the calving grounds the following spring (Gurarie et al. 2019). During cooler, windier summers, caribou remain healthier because they spend less time avoiding insects and more time eating. Unfortunately, herds that arrive late continue to be at a disadvantage because their calves have less time on the summer feeding range before migrating back to the winter range in the fall (Gurarie et al. 2019). In light of predictions for increasingly warm summers, greater insect harassment could offset any potential gains from increased forage availability or conditions, but the degree to which one outweighs the other remains unclear.
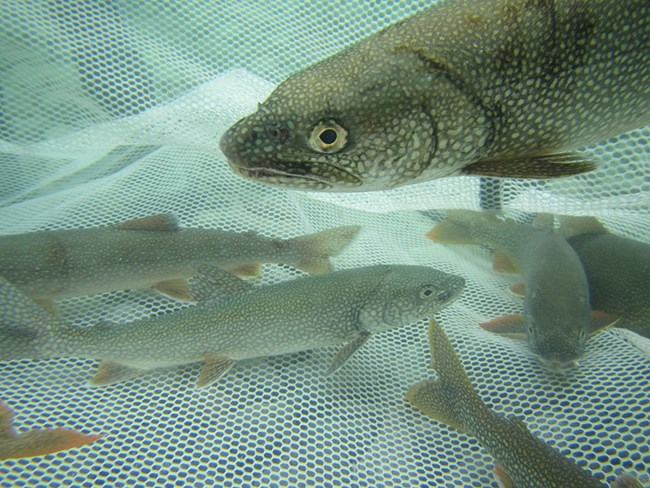
NPS/EVAN BOOHER
- Lake trout appear to benefit from warming conditions in Southwest Alaska. Warmer air temperatures mean earlier ice break-up, longer ice-free seasons, and increased zooplankton production in high-latitude lakes. Together, these conditions help lake trout, at the top of the food chain, grow larger, based on a study of seven deep, low-nutrient lakes in southwest Alaska (von Biela et al. 2020). What we don’t know yet is whether this benefit continues once lakes warm past the range of natural variation, particularly in the deeper waters normally used as thermal refugia by cold-loving species like lake trout.
- A longer growing season could mean more food for bears. With a warmer climate and longer growing season, there may be more food available for bears, especially in resource-limited places like the Arctic. A study that looked at brown bear body size and condition, and number of cubs produced between 1977 and 2016, found that food availability is important to bear health and survival, but that the effects are variable by year and location (Hilderbrand et al. 2019). As the climate warms, increased primary productivity increases populations of small mammals, like ground squirrels, which are an important food for bears (Wheeler et al. 2015). Generalizations and extrapolations over longer periods of time or geographic area are less helpful to wildlife managers than understanding local conditions and local food sources (Hilderbrand et al. 2019). But bears are omnivores and are very flexible in their food sources and their behaviors, which will likely allow them to adapt to a changing climate.

NPS/DAN YOUNG
Many species are navigating complex interactions of climate-driven habitat changes.
Changes from a warming climate are variable. A number of species experience positive and negative impacts from a changing climate and we are still teasing out these effects based on our science and long-term monitoring.
- Salmon may adapt to warming climate, if they have cold-water refugia. For Pacific salmon species, key life stage transitions depend on variables that are affected by climate warming. For example, hatching from eggs and emergence from gravel, depend on water temperature and the timing of reproduction (Beacham and Murray 1990). In a study of 25 sockeye salmon populations from the Bristol Bay region, hatching and emergence estimates spanned broad time periods: from September of the year of spawning to June of the following year for hatching, and from December of the year of spawning to August of the following year for emergence (Sparks et al. 2018). These broad time periods indicate that both hatch and emergence timing vary more widely than spawning timing, even for populations sharing the same nursery lake. The wide variation in hatching and emergence timing among populations may serve to buffer Bristol Bay sockeye salmon, as a whole, from climate change (Sparks et al. 2018). Furthermore, projections of future water temperatures through 2099 suggest that hatch timing could shift earlier by 16 to 60 days. Less known is whether spawn timing and food availability will shift in response to climate change, too.
- Shrub-adapted and forest-adapted songbirds respond to weather and climate changes differently. Weather varies from year to year and climate is the long-term trend of weather patterns. Songbirds, like many species, are impacted by both short-term weather and long-term climate. Weather and climate can have complex and sometimes conflicting effects on songbirds and all songbird species don’t respond in the same way. For example, songbirds that use shrub habitats increased their numbers in the year following a warm nesting season, but over a long-term warming climate trend, decreased in number and shifted upslope as trees and shrubs also shifted to higher elevations (Mizel et al. 2016). Overall, the temperature during the previous year’s nesting period had the greatest short-term impact on the number of songbirds observed (1995-2019). The amount of rain and snow, and the timing of snowmelt, affected different songbird species in different ways, but generally, drier conditions and earlier snowmelt led to a greater abundance of songbirds (Mizel et al. 2021).
- Bald eagles produce more young when salmon run earlier. Bald eagles often rely on salmon that return to fresh waters during the breeding season, but reproductive success can be affected by climate through both direct and indirect pathways (Schmidt et al. 2020). A study in Southwest Alaska found that warmer April temperatures were associated with increased nestling production in bald eagles (Wilson et al. 2018). In contrast, along the upper Copper River in southcentral Alaska, bald eagle nest success was positively related to early season salmon runs, although warmer springs appeared to moderate the effect of salmon abundance. This perhaps counter-intuitive result is thought to be related to increased glacial melt runoff (the Copper River has large glacial inputs) which makes it more difficult for eagles to detect salmon in the silty river (Schmidt et al. 2020). This means that although warmer temperatures may be beneficial during the early part of the nestling phase, increased glacial melt in some areas may have a countervailing impact by reducing availability of salmon as food for nestlings.

- Dall’s sheep benefit from warmer winters, unless it causes a thaw. A study of sheep survival across Alaska found that adult sheep had higher survival during warmer winters, likely because they spent less of their energy reserves to stay warm (Van de Kerk et al. 2020). Springs with delayed snowmelt led to fewer lambs and overall population declines (Rattenbury et al. 2018). Lambs thrive when summers are warm and plants are abundant. This allows rapid weight gain, which prepares lambs for winter and appears to make them less vulnerable to predators like coyotes and golden eagles (Van De Kerk et al. 2020). Although both lambs and adults benefit from warmer conditions, a warming climate may negatively impact sheep. If winter temperatures increase enough to melt snow or cause freezing rain, a thick crust of ice can form, which can make it difficult or impossible for sheep to reach the plants below. Thus, sheep survival is the lowest in winters that have many thaws. So, warm winters are good for sheep, as long as it isn’t warm enough to melt snow or create ice. Recently, sheep populations in Alaska have been in decline, which suggests that the negative effects of warming during winter may be overriding the benefits of warmer weather for sheep. Sheep are also losing habitat as warmer temperatures allow shrubs to move up in elevation. This is expected to harm sheep as shrubs replace the tender plants that they prefer and provide ambush cover for predators.
- Some caribou are calving earlier to take advantage of early spring growth. Adult females tend to choose sites to deliver their calves with abundant, high-quality food and rely on the predictability of those sites year after year (Cameron et al. 2018, 2020). Studies that looked at the timing of caribou calving in Arctic Canada found that some populations of barren-ground migratory caribou and northern mountain woodland caribou were calving earlier as the climate warmed to take advantage of new growth in the spring (Davidson et al. 2020), but this trend wasn’t evident for all Arctic herds when analyzed together (Couriot et al 2023). Another study (Gurarie et al. 2019) found that the start of spring migration for caribou herds across North America is triggered at roughly the same time by large-scale, ocean-driven climate cycles. Despite a synchronized start, arrival at their respective calving grounds depends on the previous summer’s weather conditions. When the researchers compared arrival times with climate and weather data, they consistently found that caribou herds arrived at the calving grounds earlier when they experienced cool, windy conditions the previous summer: conditions with less insect harassment.
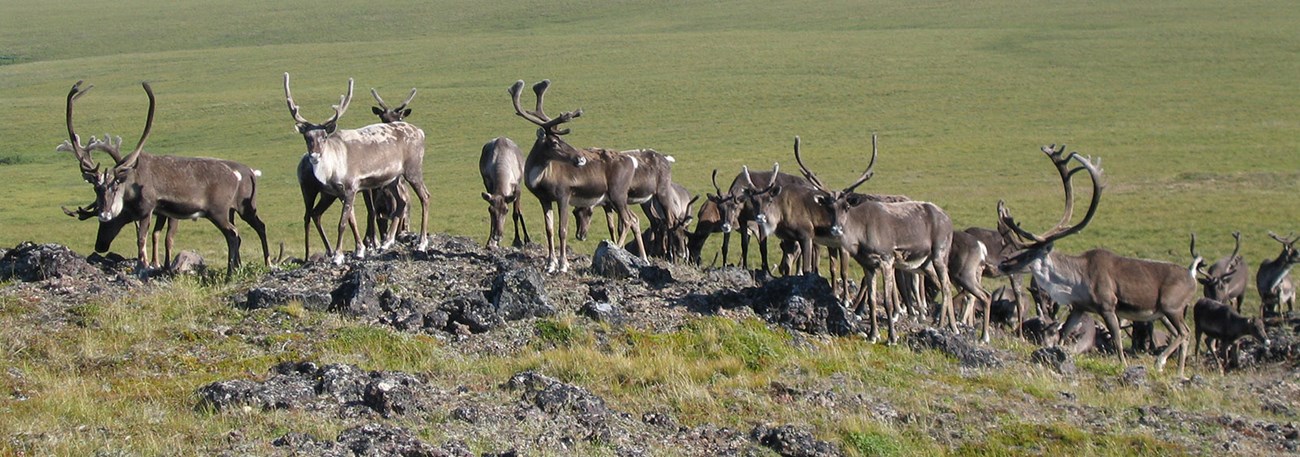
NPS/PAMELA SOUSANES
Timing of fall is later and winters have more snow.
Fall and winter weather conditions impact migration and over-winter survival of some wildlife species.
- Caribou migrate later. Colder temperatures and the first snowfalls of the winter are the main signals caribou use to initiate their fall migration (Cameron et al. 2021). Interestingly, caribou update their decisions based on local conditions. For example, if they move into a warm valley with little or no snow, they may slow down their migration and stay in that area longer before moving on. This reliance on local weather cues makes the fall migration more variable, even within a herd, because some of the herd may be in different locations and experiencing different conditions. Even with the variation in timing, the trend is that fall migration has become later for the Western Arctic Herd over the last three decades, a trend that will likely continue as temperatures warm (Cameron et al. 2021). This has disrupted local subsistence practices, some of which have been in the same place for tens of thousands of years and promises to remain a major concern for local communities that rely on caribou (Baltensperger and Joly 2019).
- Winter conditions can be important drivers for wolf populations and their prey. Because wolves are sensitive to seasonal weather conditions, they may be able to adapt to shifts in climate (Mahoney et al. 2020). Wolf survival, natality (the number of wolves added to the pack in a year), and population size increased following years with deeper snow (Borg and Schirokauer 2022). Sometimes, long-legged prey like moose and caribou can move through deep snow easier than wolves. But at some point, and especially with icing events, even they have a hard time moving in deep snow and have limited access to food resources, such as lichen for caribou. A weakened and vulnerable prey can be a boon for wolves and other predators and scavengers. However, trends toward heavier snowfall may reduce ungulate densities, thus limiting prey availability for wolves, and ultimately wolf abundance. It can take 1-2 years for wolf populations to respond to changes in primary or secondary prey (i.e., caribou and snowshoe hare, respectively). This suggests that wolves are limited by prey availability. A warming climate may mean more favorable conditions for wolves with more warm, moist winter air and greater snow depth. Conversely, conditions like warmer summers may favor ungulate population increases and ungulate body condition. Increasing numbers of caribou in good condition could mean that ungulates are less vulnerable to predation. This in turn increases search area and territory sizes for wolf packs, leading to fewer wolf packs in the same area.
How hot is too hot?
When will species hit the limits of their ability to adapt? Extreme heat and drought can cause river water to exceed temperatures that fish can stand and result in massive die-offs. Large-scale fires can result in habitat loss that can impact many species. Freeze-thaw or icing events can create food shortages and physical hardships for many species, such as caribou, moose, and small mammals. As these events become more common and widespread, wildlife will face hardships and we have yet to fully understand how they will be impacted.

NPS/MATT CAMERON
- Moose show signs of heat avoidance. Moose are another cold-adapted species that has been moving farther north as the climate warms and shrubs move into higher latitudes (Tape et al. 2016). Increased shrub abundance may lead to even more moose in the far north (Joly et al. 2012). Moose population declines have been noted in the lower-48 states connected to increasing temperatures causing heat stress (Lenarz et al. 2009, McCann et al. 2013). We were surprised to see some early signs of heat-avoidance behaviors in Alaska among moose in Gates of the Arctic National Park and Preserve—above the Arctic Circle. Moose sought out increased forest canopy cover to reduce heat stress (Jennewein et al. 2020). As fires become more extreme and burn larger areas of the Arctic, moose may have a harder time finding forest cover to get out of the heat.
- Caribou may avoid burned areas for decades after a wildfire. As wildfires increase with climate change, we found that burned areas were primarily avoided in the winter, and if caribou did wander through a burned area, they spent time where the fire was less severe and where lichens were in greater abundance relative to the rest of the burn. This pattern was due to lichens, the primary forage of wintering caribou, taking decades to recover after a burn (Joly and Cameron 2018, Joly et al. 2011). While grasses recover quickly after a fire and are a source of nutrition, caribou still mostly avoided burned areas even in the summer (Palm et al. 2022).
- Climate change will likely reduce habitat, movement corridors, and gene flow for mountain goats. Mountain goats are found in coastal mountain ranges extending from Kodiak Island to Southeast Alaska. They are dependent on alpine habitats with steep terrain nearby to escape predators. A recent study of mountain goats in Glacier Bay National Park and Preserve characterized the fine-scale genetic population structure and examined how future climate change could impact their population density (Young et al. 2022). Climate change predictions call for increased summer temperatures throughout Southeast Alaska which has been found to decrease goat survivorship (White et al. 2011). Further, warming temperatures facilitate increased tree growth at high elevations, effectively replacing alpine habitat with forest where food resources are lower and predation risk higher (White et al. 2018). Mountain goats must either adapt to this changing environment by adjusting daily activity budgets or move to more suitable habitat, which would likely mean moving higher in elevation for as far as the height of the mountain will allow. Mountain goats are able to disperse across glaciers, but due to predators, are not as successful dispersing across the low-elevation valleys that remain after glaciers retreat. At least one small sub-population of mountain goats in Glacier Bay National Park is already genetically isolated due to recent glacier retreat. Small populations are more strongly influenced by climate change due to their limited genetic diversity, and reductions in travel corridors.
Shrubs are moving north.
Shrubification, the movement of shrubland farther north, is occurring in part due to warmer soil temperatures and is bringing along moose, beavers, and songbirds. Shrubs benefit these species and potentially other species, like caribou, but as shrub habitat moves north and upward in elevation, it crowds out habitats and the species that rely on them that were already there, such as lichen-rich tundra, providing more fuel for wildland fires. Species that depend on habitats above shrub- and treeline (such as sheep and goats) will feel the pinch as vegetation changes move upward in elevation as well.
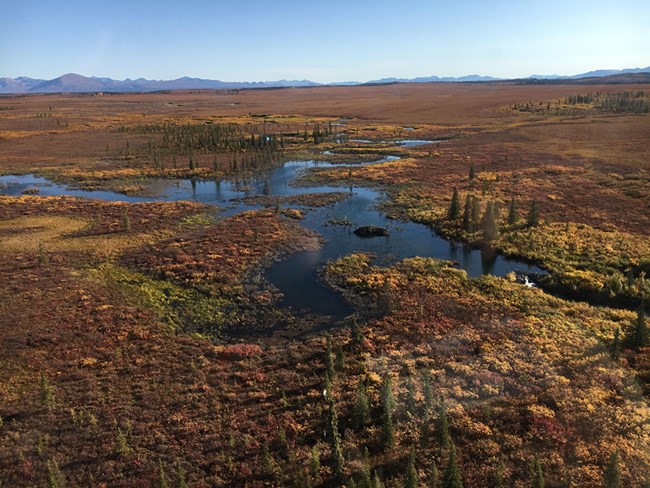
- Shrubification may benefit caribou, but benefits are offset by a loss of lichen. Across much of the caribou’s range in Alaska and Canada, expansion of deciduous shrubs is occurring northward and upward in elevation as a result of increased temperature linked to climate change. Caribou browse the preferred protein-rich leaves from shrubs that help them and their calves grow and gain weight during the summer months. But insect harassment, especially when the days are warm and the winds are calm, keeps caribou from spending more time in the shrubs and pushes them into tundra and on snow patches to avoid insects. There may be a beneficial effect of shrubs moving into higher latitudes, as this could mean more nutritious browse will be available in summer, but there is a trade-off in space for lichens that caribou depend on during the winter months (Ehlers et al. 2021, Joly et al. 2020). Another downside of shrub expansion for caribou is that it may allow for more moose, and their main predator, wolves, to move into their habitat as well as provide hiding places for caribou predators to ambush them (Joly et al. 2012).
- Beavers are moving north and contributing to climate change. Beavers, like moose, are moving north into the Arctic as shrubs move farther north. Beavers are ecosystem engineers, creating their own habitats, which can be beneficial to many species of fish, birds, and insects. But, beaver ponds have greatly increased in Arctic Alaska (Tape et al. 2022). When beaver dams create ponds and lakes, they thaw the underlying permafrost faster, which releases carbon from the soil and through the water creating methane and carbon dioxide—powerful greenhouse gases. In this way, beavers are not only responding to the warming climate, but also accelerating the effects of climate change locally (by thawing permafrost) and globally (by releasing more greenhouse gases; Tape et al. 2018).
As research shows, species are impacted by a changing climate differently depending on their sensitivities and flexibility to adapt. Warming temperatures, longer growing seasons, wetter springs, drier summers, heavier snowfalls, and other consequences of a warming climate all stimulate changes in the environment (e.g., water availability and plant communities) that ripple through the food web. Long-term monitoring, research, and modeling have given us some insights into changes that are occurring now as well as what we might see in the future.
References
Baltensperger, A. P. and K. Joly. 2019.
Using seasonal landscape models to predict space use and migratory patterns of an Arctic ungulate. Movement Ecology 7 (18).
Beacham, T. D. and C. B. Murray. 1990.
Temperature, egg size, and development of embryos and alevins of five species of Pacific salmon: A comparative analysis. Trans. Am. Fish. Soc. 119(6): 927-945.
Borg, B. L. and D. W. Schirokauer. 2022.
The role of weather and long-term prey dynamics as drivers of wolf population dynamics in a multi-prey system. Frontiers in Ecological Evolution 10: 791161.
Cameron, M. D., J. M. Eisaguirre, G. A. Breed, K. Joly, and K. Kielland. 2021.
Mechanistic movement models identify continuously updated autumn migration cues in Arctic caribou. Movement Ecology 9(54): 12 pp.
Cameron, M. D., K. Joly, G. A. Breed, C. P. H. Mulder, and K. Kielland. 2020.
Pronounced fidelity and selection for average conditions of calving area suggestive of spatial memory in a highly migratory ungulate. Frontiers in Ecology and Evolution 8: e564567.
Cameron, M. D., K. Joly, G. A. Breed, L. S. Parrett, and K. Kielland. 2018.
Movement-based methods to infer parturition events in migratory ungulates. Canadian Journal of Zoology 96: 1187-1195.
Couriot, O. H., M. D. Cameron, K. Joly, J. Adamczewski, M. W. Campbell, T. Davidson, A. Gunn, A. P. Kelly, M. Leblond, J. Williams, W. F. Fagan, A. Brose, and E. Gurarie. 2023.
Continental synchrony and local responses: Climatic effects on spatiotemporal patterns of calving in a social ungulate. Ecosphere 14(1) e.4399.
Davidson, S. C., G. Bohrer, E. Gurarie, S. LaPoint, P. J. Mahoney, ... K. Joly, ... J. P. Lawler, ...B. Mangipane, ... C. L. McIntyre, ... P. A. Owen, ... M. S. Sorum, ... et al. 2020.
Ecological insights from three decades of animal movement tracking across a changing Arctic. Science 370(6517): 712-715.
Ehlers, L., G. Coulombe, J. Herriges, T. Bentzen, M. Suitor, K. Joly, and M. Hebblewhite. 2021.
Critical summer foraging tradeoffs in a subarctic ungulate. Ecology and Evolution 11(24): 17835-17872.
Gurarie, E., M. Hebblewhite, K. Joly, A. P. Kelly, J. Adamczewski, S. C. Davidson, T. Davison, A. Gunn, M. J. Suitor, W. F. Fagan, and N. Boelman. 2019.
Tactical departures and strategic arrivals: Divergent effects of climate and weather on caribou spring migrations. Ecosphere 10(12): e02971.
Hilderbrand, G. V., K. Joly, M. S. Sorum, M. D. Cameron, and D. D. Gustine. 2019.
Brown bear (Ursus arctos) body size, condition, and productivity in the Arctic, 1977-2016. Polar Biology 42: 1-6.
Jennewein, J. S., M. Hebblewhite, P. Mahoney, S. Gilbert, A. J. H. Meddens, N. T. Boelman, K. Joly, K. Jones, K. A. Kellie, S. Brainerd, L. A. Vierling, and J. U. H. Eitel. 2020.
Behavioral modifications by a large, northern herbivore to mitigate warming conditions. Movement Ecology 8(39): 14 pp.
Joly, K., O. Couriot, M. D. Cameron, and E. Gurarie, E. 2020.
Behavioral, physiological, demographic, and ecological impacts of hematophagous and endoparasitic insects on an Arctic ungulate. Toxins 12: 334.
Joly, K., M. S. Sorum, and M. D. Cameron. 2018.
Denning ecology of wolves in east-central Alaska, 1993-2017. Arctic Institute of North America 71(4): 444.
Joly, K. and M. D. Cameron. 2018.
Early fall and late winter diets of migratory caribou in northwest Alaska. Rangifer 38(1):27-38.
Joly, K., P. A. Duffy, and T. S. Rupp. 2012.
Simulating the effects of climate change on fire regimes in Arctic biomes: Implications for caribou and moose habitat. Ecosphere 3(5): 1-18.
Joly, K., K., D. R. Klein, D. L. Verbyla, T. S. Rupp, and F. S. Chapin. 2011.
Linkages between large-scale climate patterns and the dynamics of Alaska caribou populations. Ecography. 34:345-52.
Kwon, E., E. L. Weiser, R. B. Lanctot, S. C. Brown, H. R. Gates, G. Gilchrist, S. J. Kendall, D. B. Lank, J. R. Leibezeit, L. McKinnon, E. Nol, D.C. Payer, J. Rausch, D. J. Rinella, S. T. Saalfeld, N. R. Senner, P. A. Smith, D. Ward, R. W. Wisseman, and B. K. Sandercock. 2019.
Geographic variation in the intensity of warming and phenological mismatch between Arctic shorebirds and invertebrates. Ecological Monographs 89(4): e01383.
Larsen, A. S., J. H. Schmidt, H. Stapleton, H. Kristenson, D. Betchkal, and M. F. McKenna. 2021.
Monitoring the phenology of the wood frog breeding season using bioacoustic methods. Ecological Indicators 131: 108142.
Lenarz, M. S., M. E. Nelson, M. W. Schrage, and A. J. Edwards. 2009.
Temperature mediated moose survival in northeastern Minnesota. The Journal of Wildlife Management 73(4): 503-510.
Mahoney, P. J. K. Joly, B. L. Borg, M. S. Sorum, T. A. Rinaldi, D. Saalfeld, H. Golden, A. D. M. Latham, A. P. Kelly, B. Mangipane, C. Lambert Koizumi, L. Neufeld, M. Hebblewhite, N. T. Boelman, and L. R. Prugh. 2020.
Denning phenology and reproductive success of wolves in response to climate signals. Environmental Research Letters 15(12): 125001.
McCann, N. P. R. A. Moen, and T. R. Harris. 2013.
Warm-season heat stress in moose (Alces alces). Canadian Journal of Zoology 91(12): 893-898.
Mizel, J.D., J. H. Schmidt, and C. L. McIntyre. 2021.
Climate and weather have differential effects in a high-latitude passerine community. Oecologia 195: 355-365.
Mizel, J. D., J. H. Schmidt, C. L. Mcintyre, and C. A. Roland. 2016.
Rapidly shifting elevational distributions of passerine species parallel vegetation change in the subarctic. Ecosphere 7(3): 1-15.
Palm, E. C., M. J. Suitor, K. Joly, J. D. Herriges, A. P. Kelly, D. Hervieux, K. L. M. Russell, T. W. Bentzen, N. C. Larter, and M. Hebblewhite. 2022.
Increasing fire frequency and severity will increase habitat loss for a boreal forest indicator species. Ecological Applications 32(3): e2549.
Rattenbury, K. L., J. H. Schmidt, D. K. Swanson, B. L. Borg, B. A. Mangipane, and P. J. Sousanes. 2018.
Delayed spring onset drives declines in abundance and recruitment in a mountain ungulate. Ecosphere 9(11): e02513.
Schmidt, J. H., J. Putera, and T. L. Wilson. 2020.
Direct and indirect effects of temperature and prey abundance on bald eagle reproductive dynamics. Oecologia 192: 391-401.
Shaftel, R., D. J. Rinella, E. Kwon, S. C. Brown, H. R. Gates, S. Kendall, D. B. Lank, J. R. Liebezeit, D. C. Payer, J. Rausch, S. T. Saalfeld, B. K. Sandercock, P. A. Smith, D. H. Ward, and R. B. Lanctot. 2021.
Predictors of invertebrate biomass and rate of advancement of invertebrate phenology across eight sites in the North American Arctic. Polar Biology 44: 237-257.
Sparks, M. M., J. A. Falke, T. P. Quinn, M. D. Adkison, D. E. Schindler, K. Bartz, D. Young, and P. A.H. Westley. 2018.
Influences of spawning timing, water temperature, and climatic warming on early life history phenology in western Alaska sockeye salmon. Canadian Journal of Fisheries and Aquatic Sciences 76: 123-135.
Tape, K. D. J. A. Clark, B. M. Jones, S. Kantner, B. V. Gaglioti, G. Grosse, and I. Nitze. 2022.
Expanding beaver pond distribution in Arctic Alaska, 1949 to 2019. Scientific Reports 12: 7123.
Tape, K. D., B. M. Jones, C. D. Arp, I. Nitze, and G. Grosse. 2018.
Tundra be dammed: Beaver colonization of the Arctic. Global Change Biology 24: 4478-4488.
Tape, K. D., D. D. Gustine, R. W. Ruess, L. G. Adams, and J. A. Clark. 2016.
Range expansion of moose in Arctic Alaska linked to warming and increased shrub habitat. PLOS ONE 11(7): e0160049.
Van de Kerk, M., S. Arthur, M. Bertram, B. Borg, J. Herriges, J. Lawler, B. Mangipane, C. Lambert Koizumi, B. Wendling, and L. Prugh. 2020.
Environmental influences on Dall’s sheep survival. Journal of Wildlife Management 84: 1127-1138.
von Biela, V. R., B. A. Black, D. B. Young, P. van der Sleen, K. K. Bartz, and C. E. Zimmerman. 2020.
Lake trout growth is sensitive to spring temperature in southwest Alaska lakes. Ecology of Freshwater Fish 30(1): 88-99.
Wheeler, H.C., J. D. Chipperfield, C. Roland, and J. C. Svenning. 2015.
How will the greening of the Arctic affect a common ecosystem engineer? Vegetation effects on burrowing networks and activity of arctic ground squirrels. Oecologia 178(3): 915-929.
White, K. S., G. W. Pendleton, D. Crowley, H. J. Griese, K. J. Hundertmark, T. McDonough, L. 534 Nichols, M. Robus, C. A. Smith, and J. W. Schoen. 2011.
Mountain goat survival in coastal 535 Alaska: Effects of age, sex, and climate. Journal of Wildlife Management 75: 1731-1744.
White, K. S., D. P. Gregovich, and T. Levi. 2018.
Projecting the future of an alpine ungulate under climate change scenarios. Global Change Biology 24: 1136–1149.
Wilson, T., J. Schmidt, B. Mangipane, R. Kolstrom, and K. Bartz. 2018.
Nest use dynamics of an undisturbed population of bald eagles in Lake Clark National Park and Preserve, Alaska. Ecology and Evolution 8(15): 7346-7354.
Young, K. B., T. M. Lewis, K. S. White, and A. B. A. Shafer. 2022.
Quantifying the effects of recent glacial history and future climate change on a unique population of mountain goats. Biological Conservation 272(109631): 1-8.
Last updated: September 6, 2023